Jan 14, 2025
Jan 14, 2025
Batteries 101: How Do Batteries Work?
Batteries 101: How Do Batteries Work?

To kick off 2025, we’re starting a new series of blog posts called “Batteries 101”, where we’ll dive into the fundamentals of lithium-ion batteries. Whether you’re new to battery science or just looking to brush up on the basics, this series will guide you through the inner workings of these devices that power our everyday lives—from phones and laptops to electric vehicles. In this first post, we’ll start with the basics: what’s inside a lithium-ion battery and how it works.
If you were to open a lithium-ion battery (please don’t try this at home under any circumstances), you’d find that it contains either a very long and thin sheet rolled up (in cylindrical or prismatic batteries) or several thin sheets stacked on top of one another (in pouch batteries). Looking closer, we’d notice that these sheets consist of several layers stacked together:
Positive Electrode: Often referred to as the cathode (more on this later). This is a porous layer typically made of lithium-metal oxide particles (e.g., lithium cobalt oxide or lithium iron phosphate) “glued” together by some additives.
Negative Electrode: Often called the anode, it is similar to the positive electrode but typically made of graphite.
Separator: A thin, porous membrane that keeps the positive and negative electrodes apart to prevent short circuits, while allowing ions to pass through.
Electrolyte: A liquid or gel that fills the pores within the electrodes and separator, facilitating the movement of lithium ions. It usually consists of a lithium salt dissolved in an organic solvent.
Current Collectors: Thin metal foils (typically aluminum for the positive electrode and copper for the negative one) that connect the electrodes to the external circuit.

Wikimedia commons: battery with a polymer separator
This combination of components, often referred to as a cell, forms the basic electrochemical unit capable of storing and delivering energy.
At the heart of a battery’s operation lies the movement of lithium ions and electrons between the electrodes. Here’s a simplified explanation of the process:
When Fully Charged: Lithium ions are stored (or "intercalated") within the layers of the negative electrode. This is the battery’s high-energy state, ready to deliver power.
Discharge: When the battery is connected to a device, the external circuit is closed, and a chemical reaction occurs. Lithium ions leave the negative electrode (deintercalate) and travel through the electrolyte and separator to the positive electrode. Simultaneously, electrons are released from the negative electrode, travel through the external circuit (powering the device), and re-enter the battery at the positive electrode. Here, the lithium ions and electrons combine to form intercalated lithium in the positive electrode.
Charge: By applying an external voltage to the battery, the process is reversed. Lithium ions move back to the negative electrode, restoring the battery to its high-energy state.
This reversible movement of lithium ions and electrons is what makes lithium-ion batteries rechargeable. Of course, the process also involves additional phenomena such as gas formation, electrode swelling, and solid-electrolyte interphase growth, but let’s not get ahead of ourselves.
Now that we’ve covered the basics, we can dive deeper into some key concepts introduced here. In the upcoming posts, we’ll clarify the difference between negative and positive electrodes (and why they’re often called anode and cathode), explain what open-circuit potentials are, and explore interface kinetic equations like Butler-Volmer and Tafel. We’ll even discuss phenomena like hysteresis and battery degradation, which strongly influence battery performance.
Stay tuned for the next post, where we’ll kick things off by unpacking the terminology of anodes and cathodes—and why their names depend on whether the battery is charging or discharging!
To kick off 2025, we’re starting a new series of blog posts called “Batteries 101”, where we’ll dive into the fundamentals of lithium-ion batteries. Whether you’re new to battery science or just looking to brush up on the basics, this series will guide you through the inner workings of these devices that power our everyday lives—from phones and laptops to electric vehicles. In this first post, we’ll start with the basics: what’s inside a lithium-ion battery and how it works.
If you were to open a lithium-ion battery (please don’t try this at home under any circumstances), you’d find that it contains either a very long and thin sheet rolled up (in cylindrical or prismatic batteries) or several thin sheets stacked on top of one another (in pouch batteries). Looking closer, we’d notice that these sheets consist of several layers stacked together:
Positive Electrode: Often referred to as the cathode (more on this later). This is a porous layer typically made of lithium-metal oxide particles (e.g., lithium cobalt oxide or lithium iron phosphate) “glued” together by some additives.
Negative Electrode: Often called the anode, it is similar to the positive electrode but typically made of graphite.
Separator: A thin, porous membrane that keeps the positive and negative electrodes apart to prevent short circuits, while allowing ions to pass through.
Electrolyte: A liquid or gel that fills the pores within the electrodes and separator, facilitating the movement of lithium ions. It usually consists of a lithium salt dissolved in an organic solvent.
Current Collectors: Thin metal foils (typically aluminum for the positive electrode and copper for the negative one) that connect the electrodes to the external circuit.

Wikimedia commons: battery with a polymer separator
This combination of components, often referred to as a cell, forms the basic electrochemical unit capable of storing and delivering energy.
At the heart of a battery’s operation lies the movement of lithium ions and electrons between the electrodes. Here’s a simplified explanation of the process:
When Fully Charged: Lithium ions are stored (or "intercalated") within the layers of the negative electrode. This is the battery’s high-energy state, ready to deliver power.
Discharge: When the battery is connected to a device, the external circuit is closed, and a chemical reaction occurs. Lithium ions leave the negative electrode (deintercalate) and travel through the electrolyte and separator to the positive electrode. Simultaneously, electrons are released from the negative electrode, travel through the external circuit (powering the device), and re-enter the battery at the positive electrode. Here, the lithium ions and electrons combine to form intercalated lithium in the positive electrode.
Charge: By applying an external voltage to the battery, the process is reversed. Lithium ions move back to the negative electrode, restoring the battery to its high-energy state.
This reversible movement of lithium ions and electrons is what makes lithium-ion batteries rechargeable. Of course, the process also involves additional phenomena such as gas formation, electrode swelling, and solid-electrolyte interphase growth, but let’s not get ahead of ourselves.
Now that we’ve covered the basics, we can dive deeper into some key concepts introduced here. In the upcoming posts, we’ll clarify the difference between negative and positive electrodes (and why they’re often called anode and cathode), explain what open-circuit potentials are, and explore interface kinetic equations like Butler-Volmer and Tafel. We’ll even discuss phenomena like hysteresis and battery degradation, which strongly influence battery performance.
Stay tuned for the next post, where we’ll kick things off by unpacking the terminology of anodes and cathodes—and why their names depend on whether the battery is charging or discharging!
To kick off 2025, we’re starting a new series of blog posts called “Batteries 101”, where we’ll dive into the fundamentals of lithium-ion batteries. Whether you’re new to battery science or just looking to brush up on the basics, this series will guide you through the inner workings of these devices that power our everyday lives—from phones and laptops to electric vehicles. In this first post, we’ll start with the basics: what’s inside a lithium-ion battery and how it works.
If you were to open a lithium-ion battery (please don’t try this at home under any circumstances), you’d find that it contains either a very long and thin sheet rolled up (in cylindrical or prismatic batteries) or several thin sheets stacked on top of one another (in pouch batteries). Looking closer, we’d notice that these sheets consist of several layers stacked together:
Positive Electrode: Often referred to as the cathode (more on this later). This is a porous layer typically made of lithium-metal oxide particles (e.g., lithium cobalt oxide or lithium iron phosphate) “glued” together by some additives.
Negative Electrode: Often called the anode, it is similar to the positive electrode but typically made of graphite.
Separator: A thin, porous membrane that keeps the positive and negative electrodes apart to prevent short circuits, while allowing ions to pass through.
Electrolyte: A liquid or gel that fills the pores within the electrodes and separator, facilitating the movement of lithium ions. It usually consists of a lithium salt dissolved in an organic solvent.
Current Collectors: Thin metal foils (typically aluminum for the positive electrode and copper for the negative one) that connect the electrodes to the external circuit.

Wikimedia commons: battery with a polymer separator
This combination of components, often referred to as a cell, forms the basic electrochemical unit capable of storing and delivering energy.
At the heart of a battery’s operation lies the movement of lithium ions and electrons between the electrodes. Here’s a simplified explanation of the process:
When Fully Charged: Lithium ions are stored (or "intercalated") within the layers of the negative electrode. This is the battery’s high-energy state, ready to deliver power.
Discharge: When the battery is connected to a device, the external circuit is closed, and a chemical reaction occurs. Lithium ions leave the negative electrode (deintercalate) and travel through the electrolyte and separator to the positive electrode. Simultaneously, electrons are released from the negative electrode, travel through the external circuit (powering the device), and re-enter the battery at the positive electrode. Here, the lithium ions and electrons combine to form intercalated lithium in the positive electrode.
Charge: By applying an external voltage to the battery, the process is reversed. Lithium ions move back to the negative electrode, restoring the battery to its high-energy state.
This reversible movement of lithium ions and electrons is what makes lithium-ion batteries rechargeable. Of course, the process also involves additional phenomena such as gas formation, electrode swelling, and solid-electrolyte interphase growth, but let’s not get ahead of ourselves.
Now that we’ve covered the basics, we can dive deeper into some key concepts introduced here. In the upcoming posts, we’ll clarify the difference between negative and positive electrodes (and why they’re often called anode and cathode), explain what open-circuit potentials are, and explore interface kinetic equations like Butler-Volmer and Tafel. We’ll even discuss phenomena like hysteresis and battery degradation, which strongly influence battery performance.
Stay tuned for the next post, where we’ll kick things off by unpacking the terminology of anodes and cathodes—and why their names depend on whether the battery is charging or discharging!
To kick off 2025, we’re starting a new series of blog posts called “Batteries 101”, where we’ll dive into the fundamentals of lithium-ion batteries. Whether you’re new to battery science or just looking to brush up on the basics, this series will guide you through the inner workings of these devices that power our everyday lives—from phones and laptops to electric vehicles. In this first post, we’ll start with the basics: what’s inside a lithium-ion battery and how it works.
If you were to open a lithium-ion battery (please don’t try this at home under any circumstances), you’d find that it contains either a very long and thin sheet rolled up (in cylindrical or prismatic batteries) or several thin sheets stacked on top of one another (in pouch batteries). Looking closer, we’d notice that these sheets consist of several layers stacked together:
Positive Electrode: Often referred to as the cathode (more on this later). This is a porous layer typically made of lithium-metal oxide particles (e.g., lithium cobalt oxide or lithium iron phosphate) “glued” together by some additives.
Negative Electrode: Often called the anode, it is similar to the positive electrode but typically made of graphite.
Separator: A thin, porous membrane that keeps the positive and negative electrodes apart to prevent short circuits, while allowing ions to pass through.
Electrolyte: A liquid or gel that fills the pores within the electrodes and separator, facilitating the movement of lithium ions. It usually consists of a lithium salt dissolved in an organic solvent.
Current Collectors: Thin metal foils (typically aluminum for the positive electrode and copper for the negative one) that connect the electrodes to the external circuit.

Wikimedia commons: battery with a polymer separator
This combination of components, often referred to as a cell, forms the basic electrochemical unit capable of storing and delivering energy.
At the heart of a battery’s operation lies the movement of lithium ions and electrons between the electrodes. Here’s a simplified explanation of the process:
When Fully Charged: Lithium ions are stored (or "intercalated") within the layers of the negative electrode. This is the battery’s high-energy state, ready to deliver power.
Discharge: When the battery is connected to a device, the external circuit is closed, and a chemical reaction occurs. Lithium ions leave the negative electrode (deintercalate) and travel through the electrolyte and separator to the positive electrode. Simultaneously, electrons are released from the negative electrode, travel through the external circuit (powering the device), and re-enter the battery at the positive electrode. Here, the lithium ions and electrons combine to form intercalated lithium in the positive electrode.
Charge: By applying an external voltage to the battery, the process is reversed. Lithium ions move back to the negative electrode, restoring the battery to its high-energy state.
This reversible movement of lithium ions and electrons is what makes lithium-ion batteries rechargeable. Of course, the process also involves additional phenomena such as gas formation, electrode swelling, and solid-electrolyte interphase growth, but let’s not get ahead of ourselves.
Now that we’ve covered the basics, we can dive deeper into some key concepts introduced here. In the upcoming posts, we’ll clarify the difference between negative and positive electrodes (and why they’re often called anode and cathode), explain what open-circuit potentials are, and explore interface kinetic equations like Butler-Volmer and Tafel. We’ll even discuss phenomena like hysteresis and battery degradation, which strongly influence battery performance.
Stay tuned for the next post, where we’ll kick things off by unpacking the terminology of anodes and cathodes—and why their names depend on whether the battery is charging or discharging!
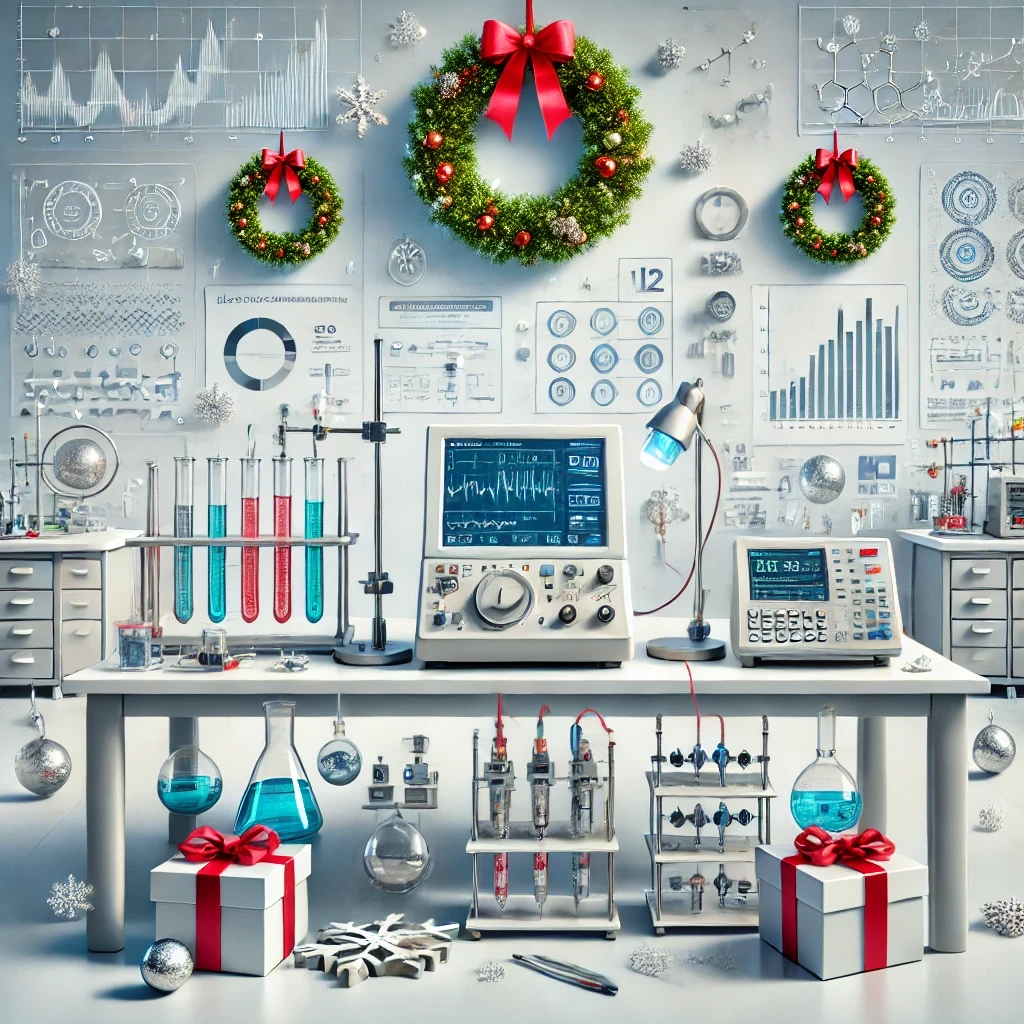
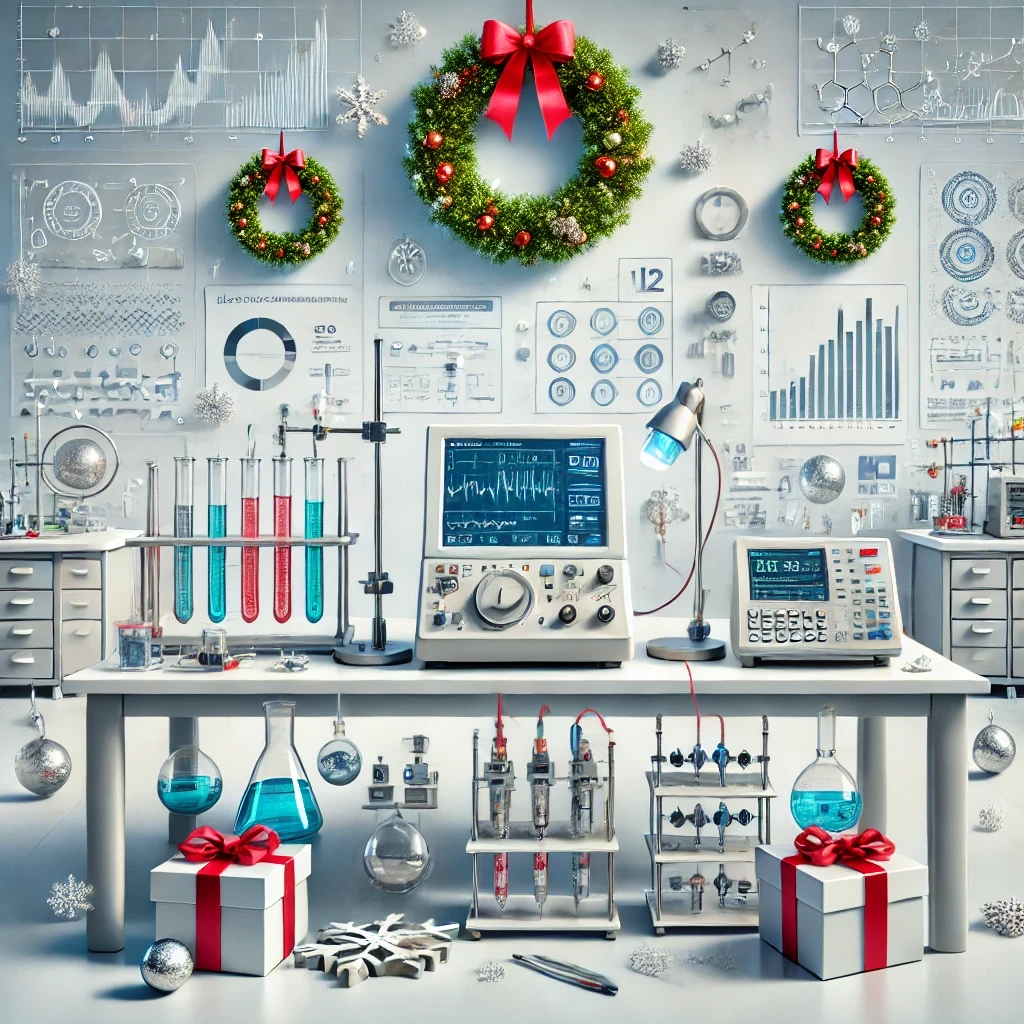
12 Days of Electrochemical Testing
To celebrate the holiday season and the re-release of Ionworks Studio, we featured "12 (business) days of electrochemical testing". Each day we pick a test, give a little bit of information about it, and show you how to run it in Ionworks. 🔋 🎄
Dec 17, 2024
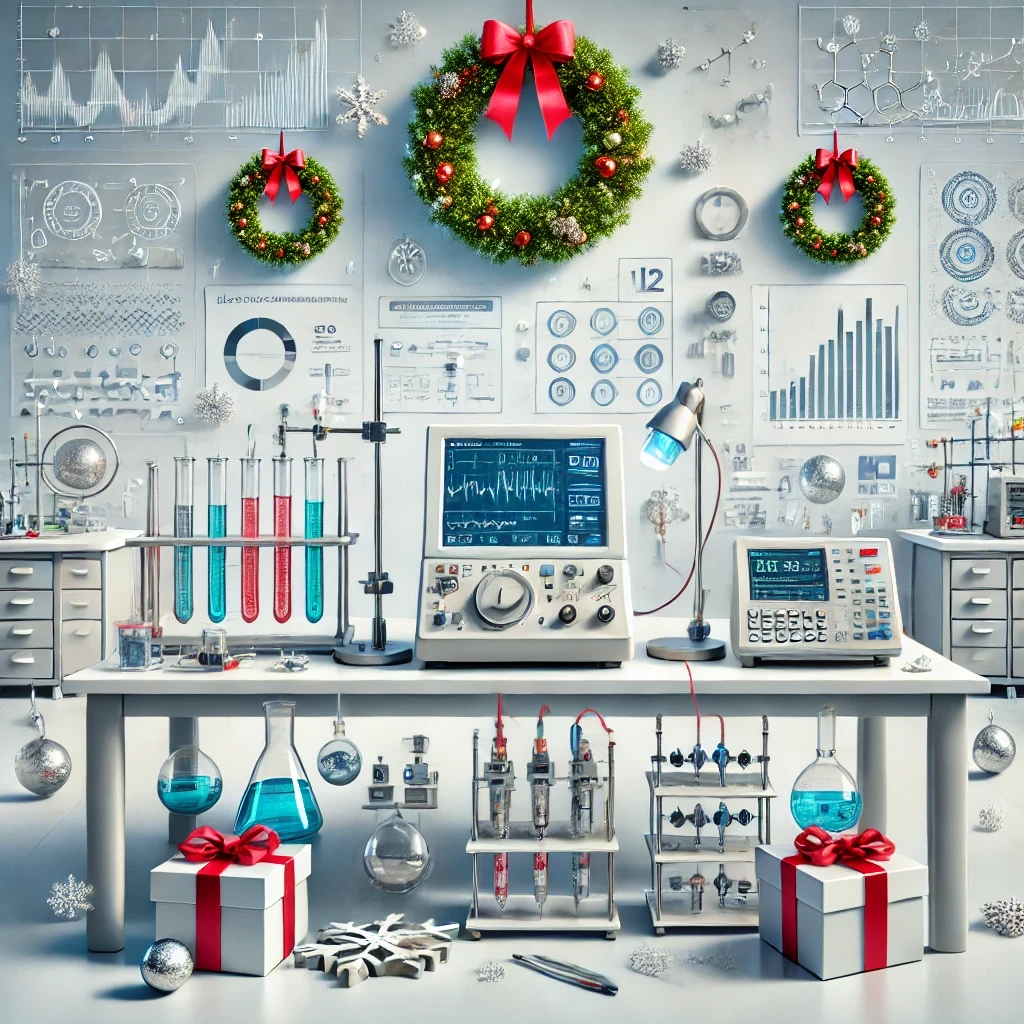
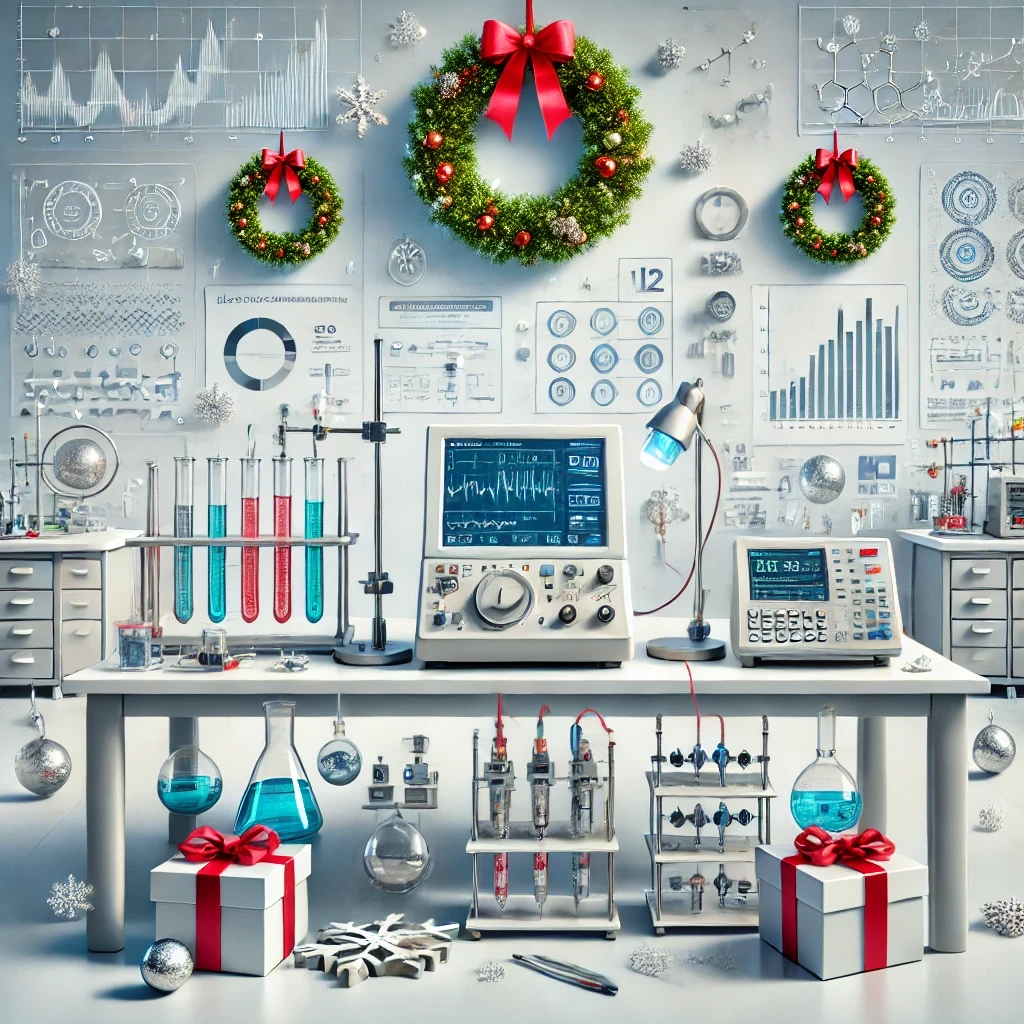
12 Days of Electrochemical Testing
To celebrate the holiday season and the re-release of Ionworks Studio, we featured "12 (business) days of electrochemical testing". Each day we pick a test, give a little bit of information about it, and show you how to run it in Ionworks. 🔋 🎄
Dec 17, 2024
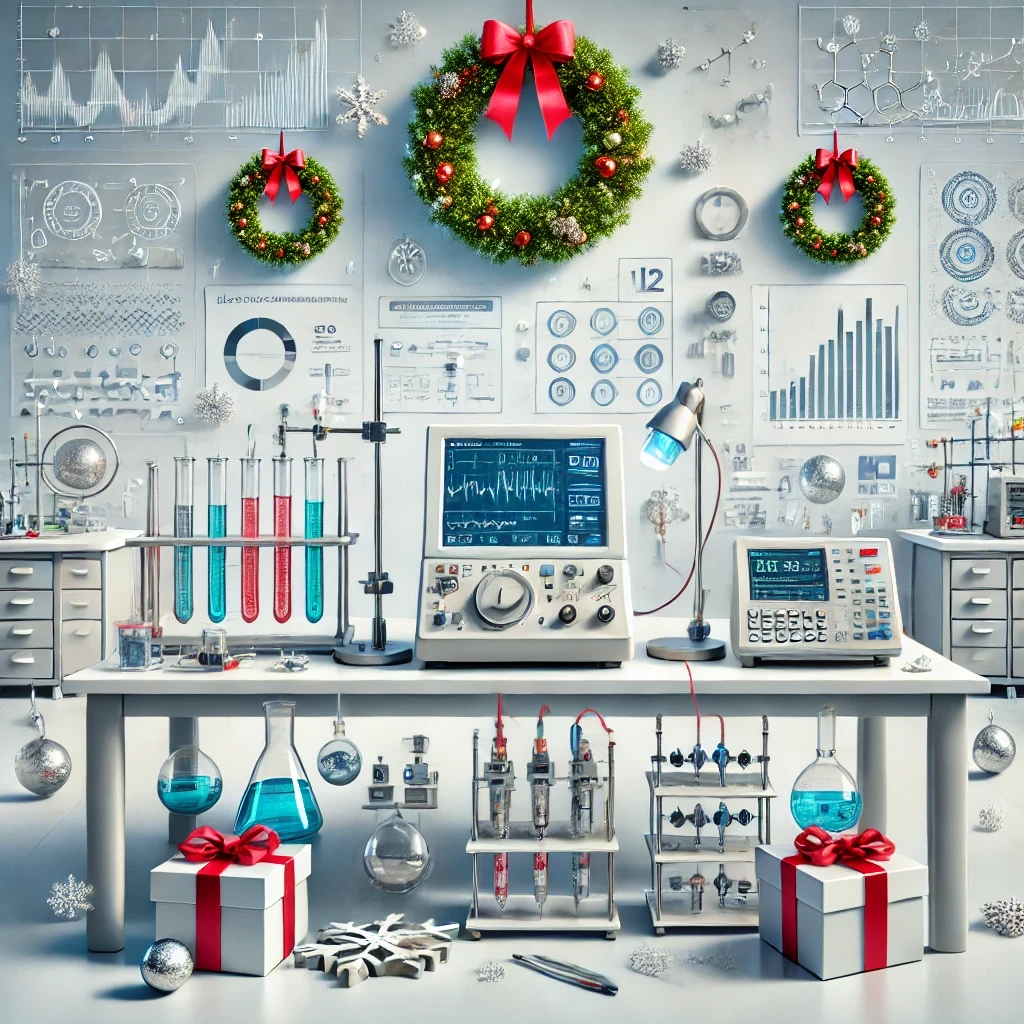
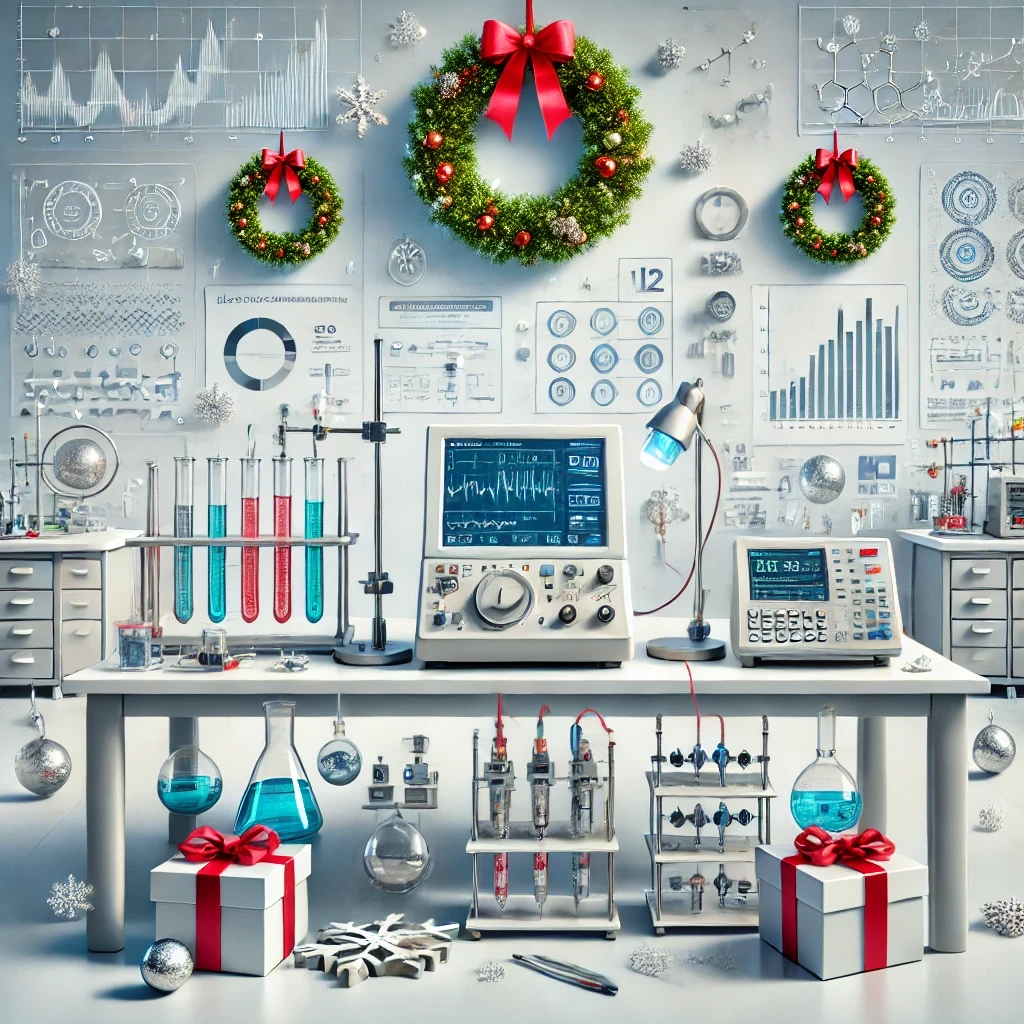
12 Days of Electrochemical Testing
To celebrate the holiday season and the re-release of Ionworks Studio, we featured "12 (business) days of electrochemical testing". Each day we pick a test, give a little bit of information about it, and show you how to run it in Ionworks. 🔋 🎄
Dec 17, 2024
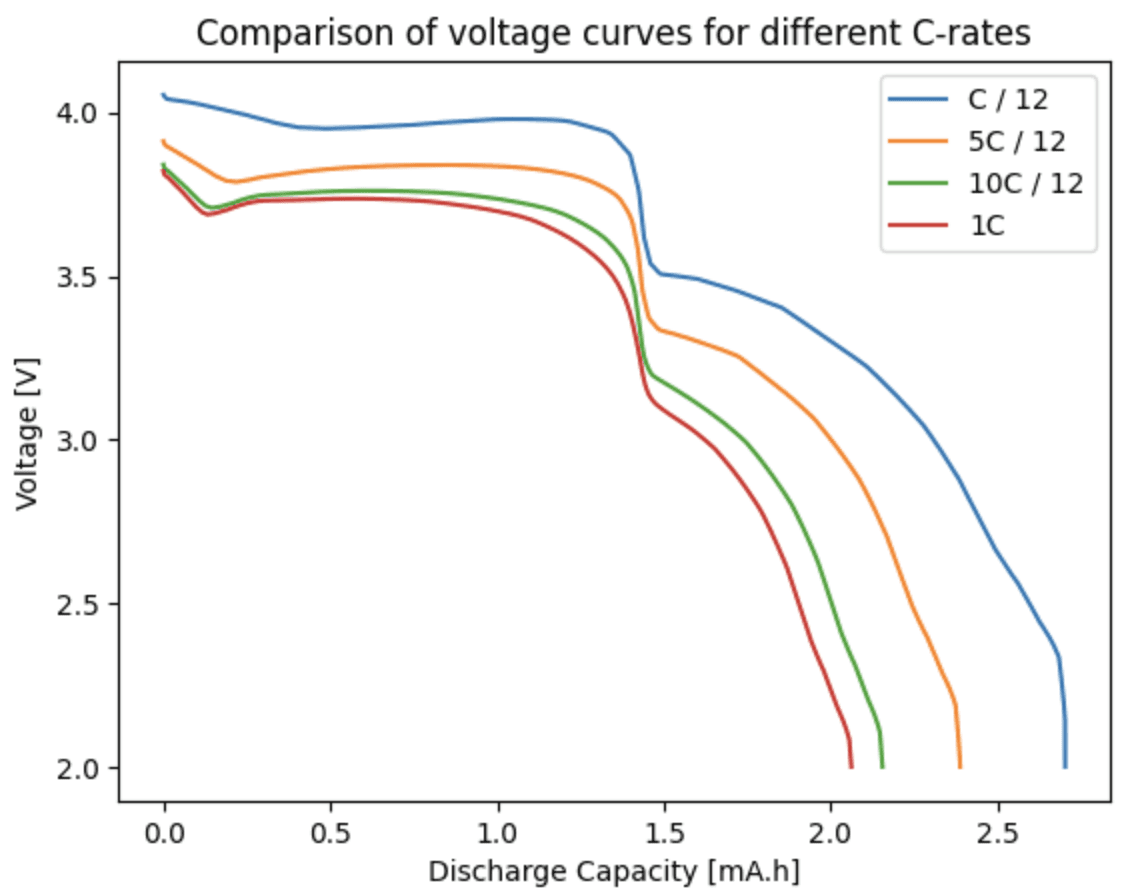
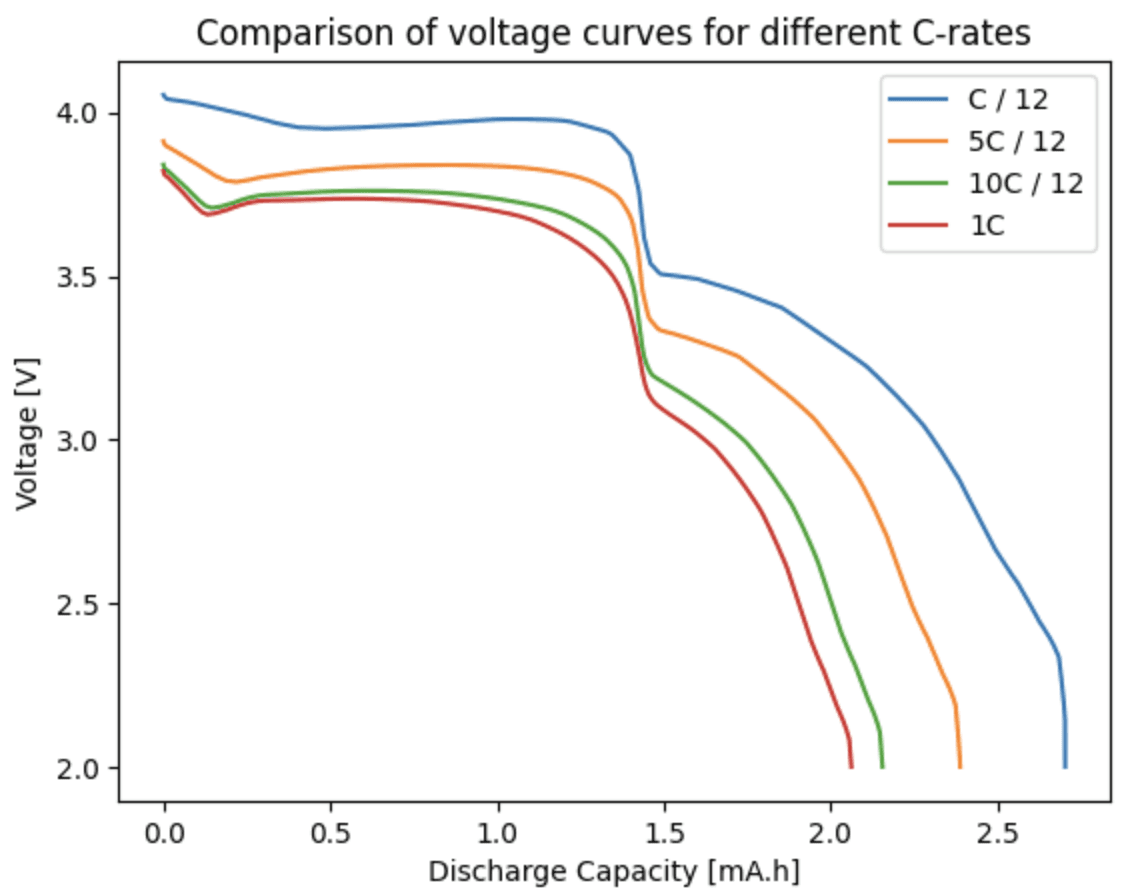
Sodium Ion Battery Model now available in PyBaMM!
This blog post explores the history of SIBs, the intricacies of the new PyBaMM model, and the exciting possibilities it unlocks for the future of energy storage.
Nov 12, 2024
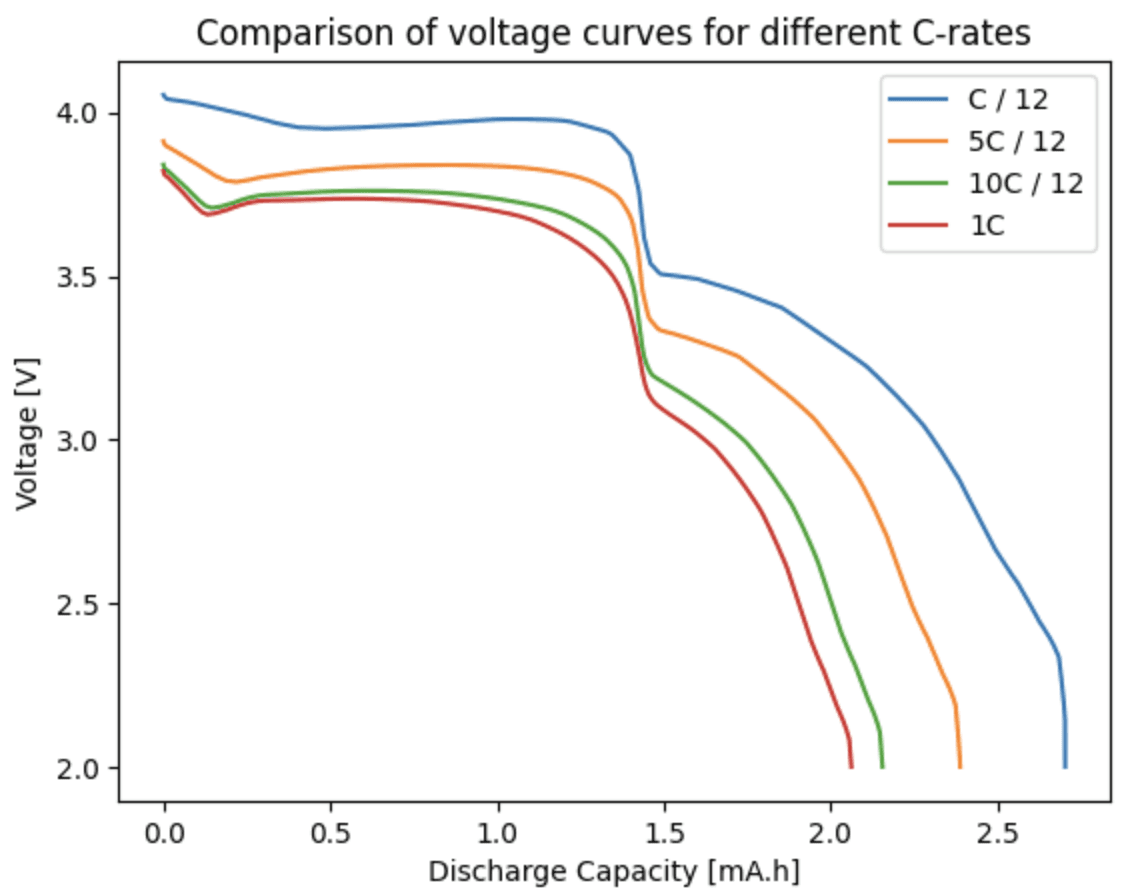
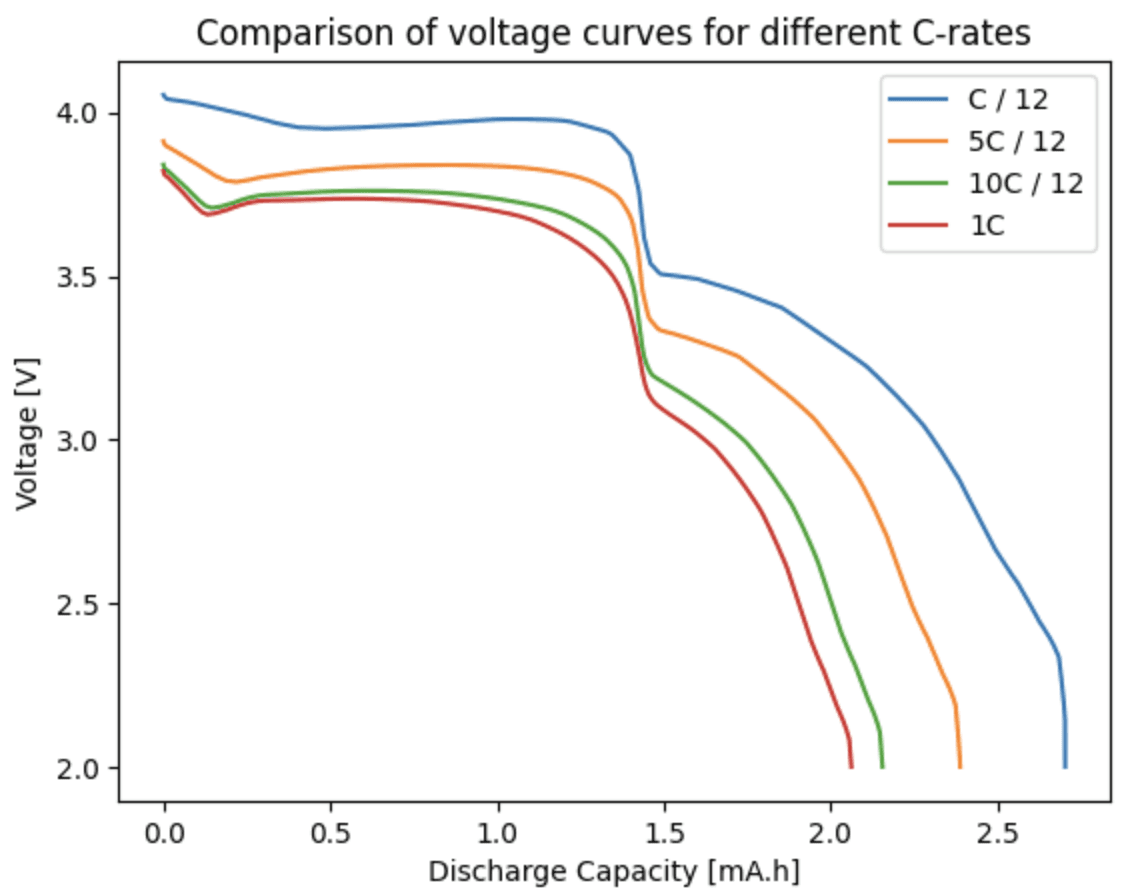
Sodium Ion Battery Model now available in PyBaMM!
This blog post explores the history of SIBs, the intricacies of the new PyBaMM model, and the exciting possibilities it unlocks for the future of energy storage.
Nov 12, 2024
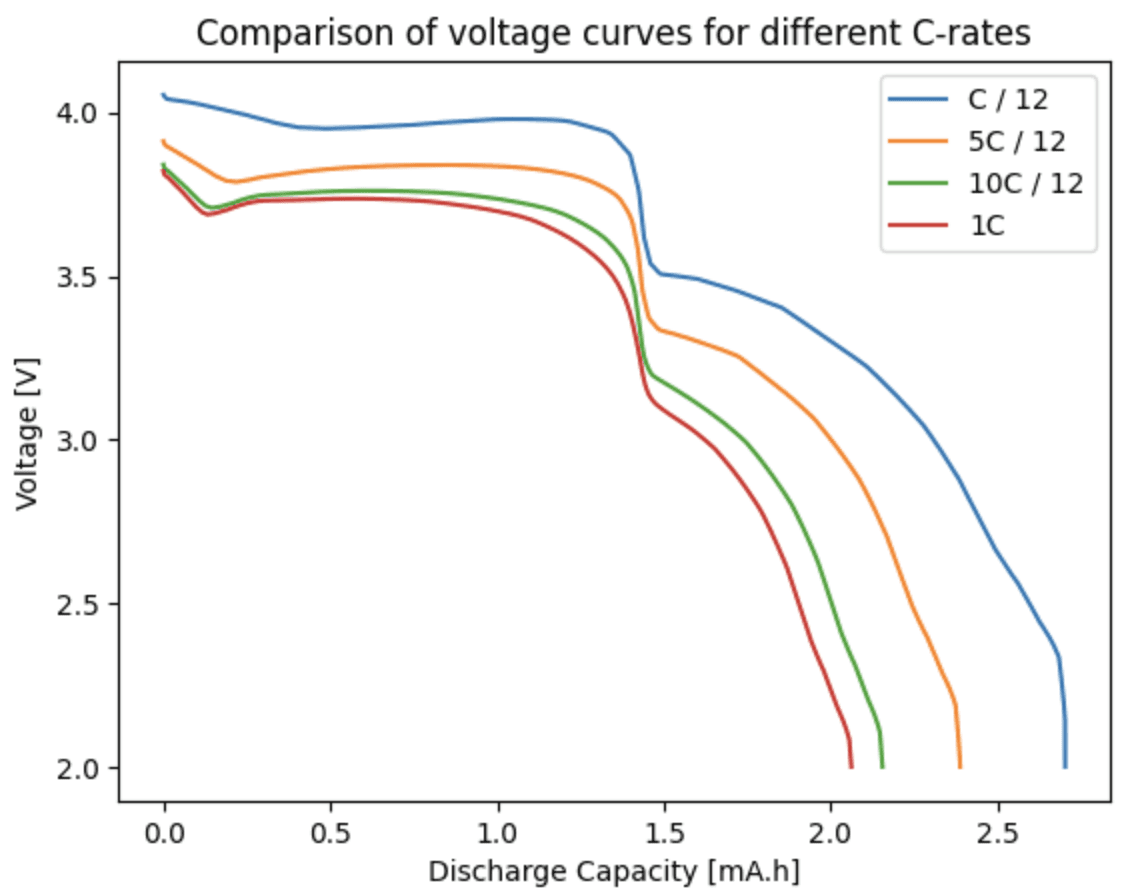
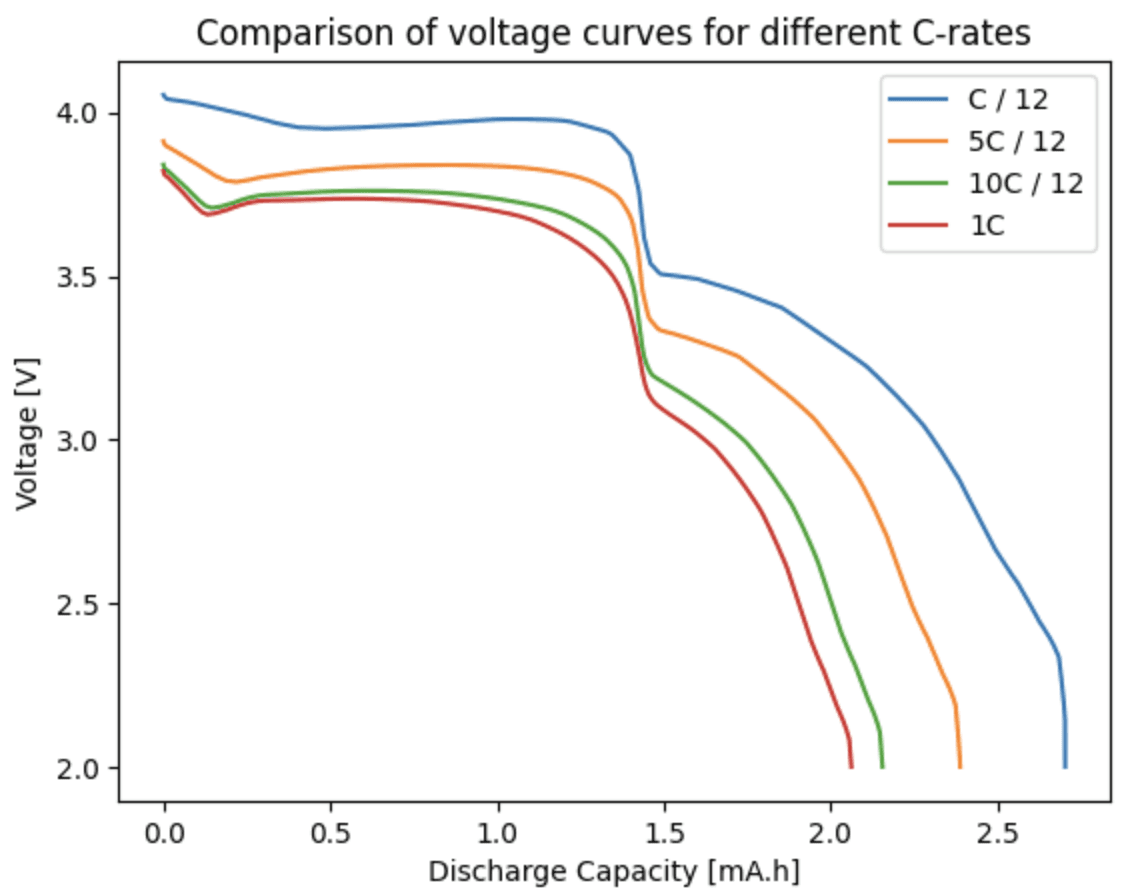
Sodium Ion Battery Model now available in PyBaMM!
This blog post explores the history of SIBs, the intricacies of the new PyBaMM model, and the exciting possibilities it unlocks for the future of energy storage.
Nov 12, 2024
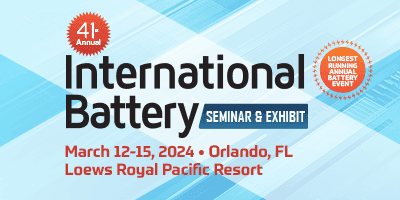
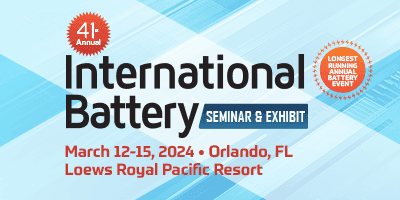
Ionworks Presents at International Battery Seminar
Ionworks CEO Valentin Sulzer presents at the International Battery Seminar in Florida
Mar 12, 2024
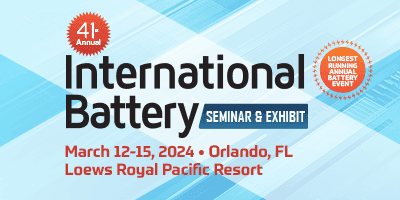
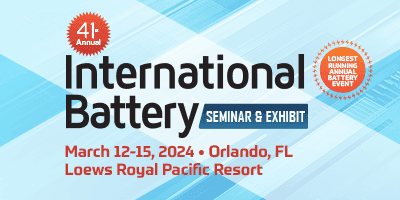
Ionworks Presents at International Battery Seminar
Ionworks CEO Valentin Sulzer presents at the International Battery Seminar in Florida
Mar 12, 2024
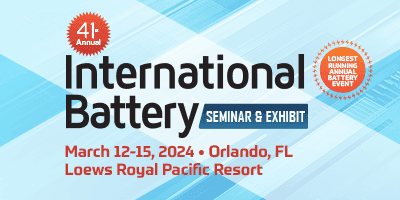
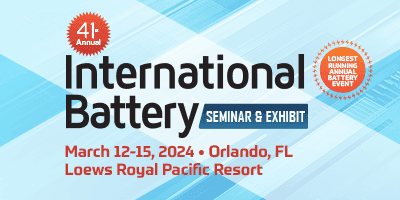
Ionworks Presents at International Battery Seminar
Ionworks CEO Valentin Sulzer presents at the International Battery Seminar in Florida
Mar 12, 2024
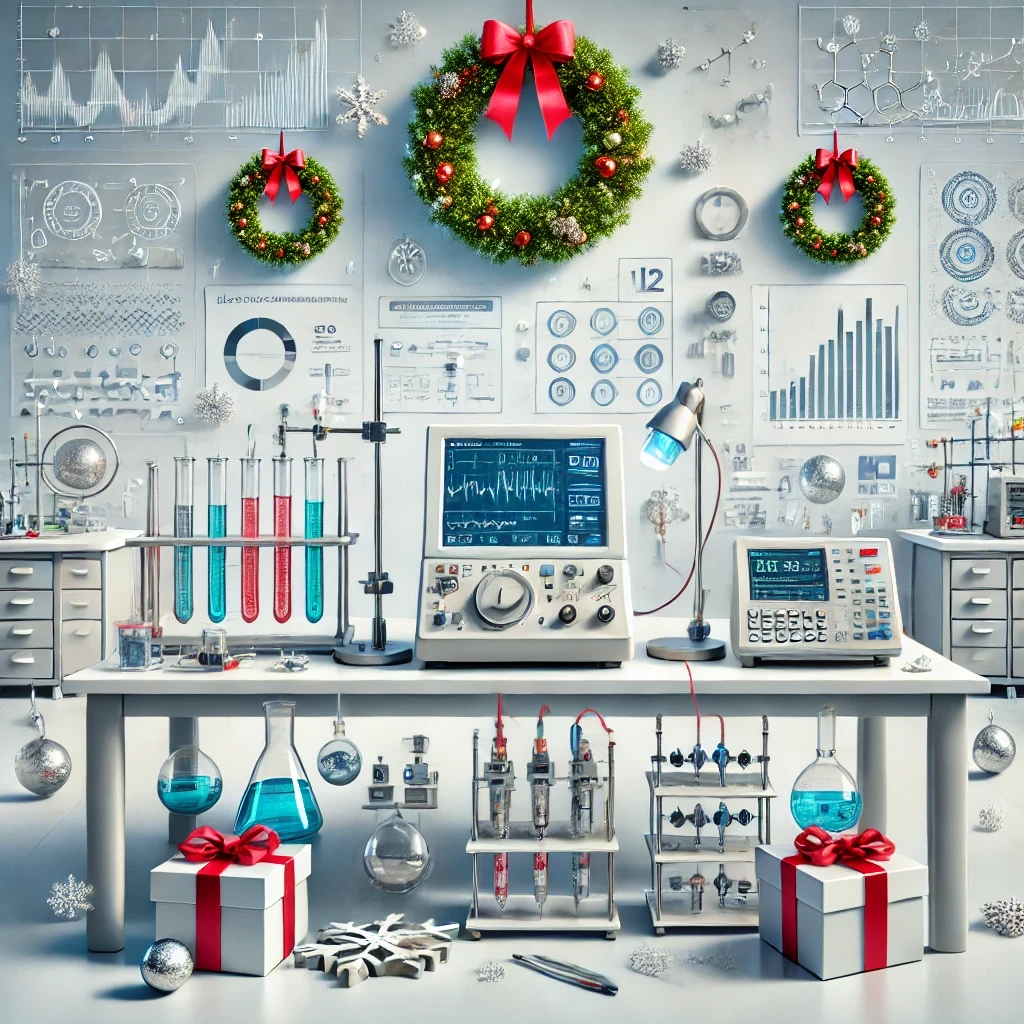
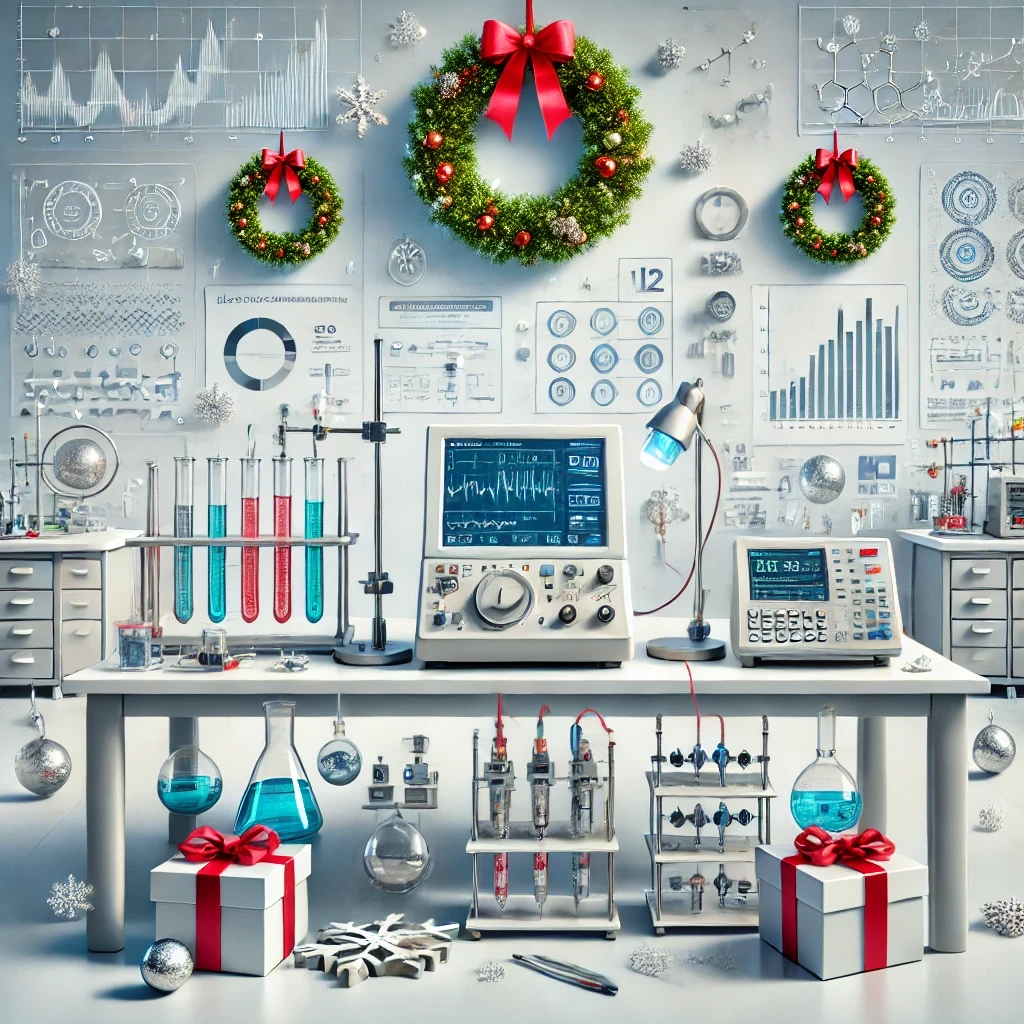
12 Days of Electrochemical Testing
To celebrate the holiday season and the re-release of Ionworks Studio, we featured "12 (business) days of electrochemical testing". Each day we pick a test, give a little bit of information about it, and show you how to run it in Ionworks. 🔋 🎄
Dec 17, 2024
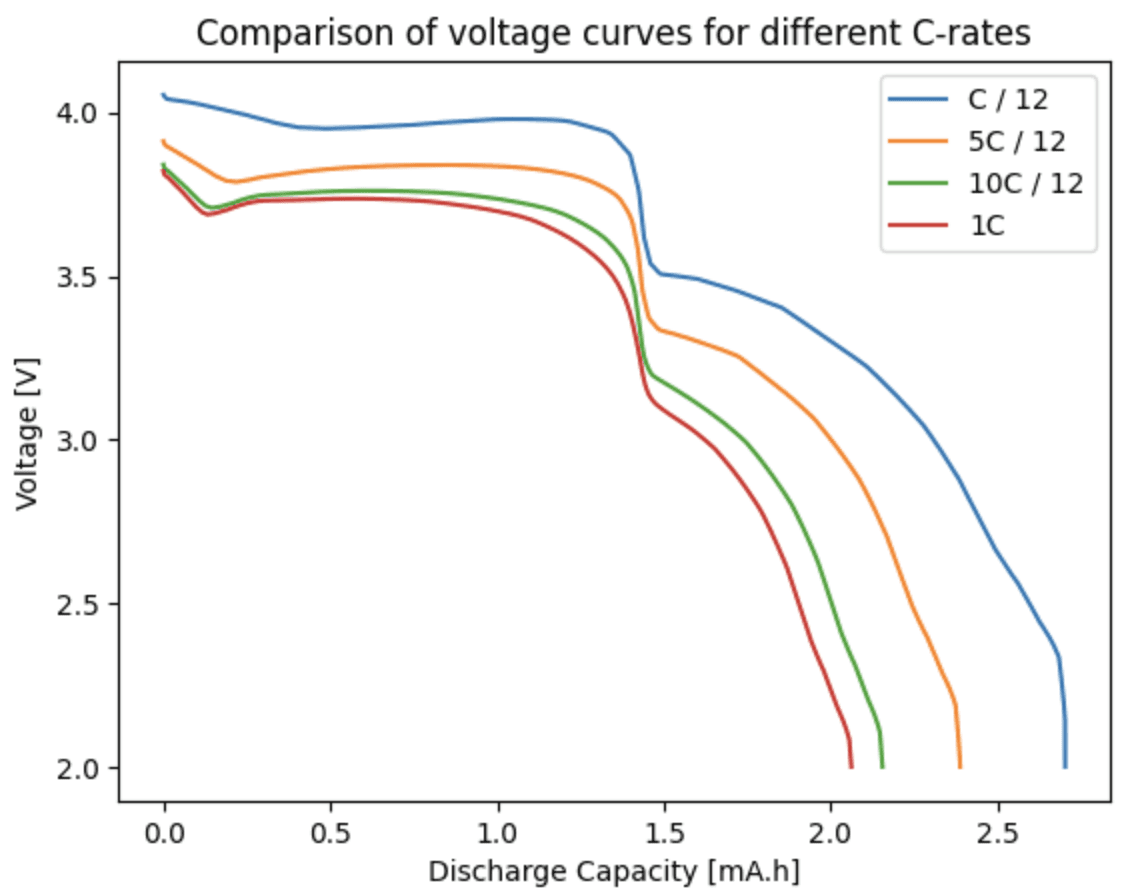
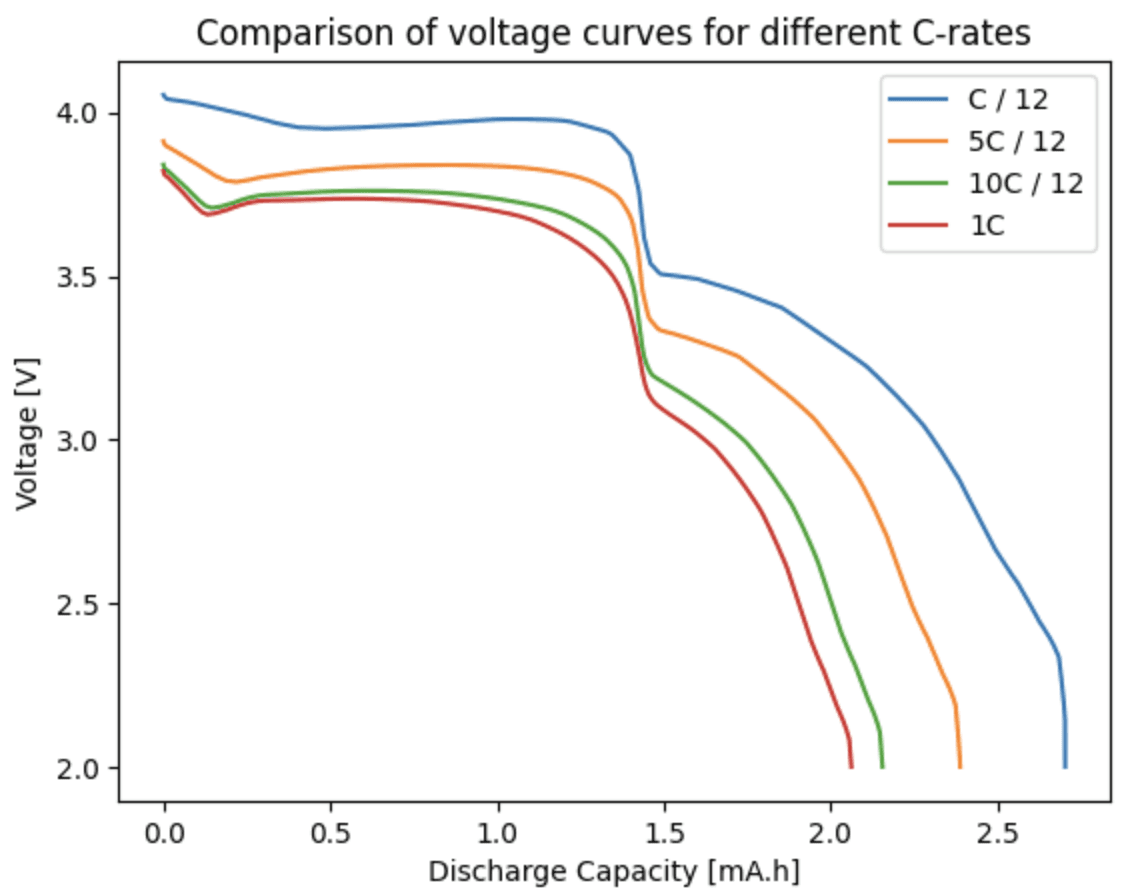
Sodium Ion Battery Model now available in PyBaMM!
This blog post explores the history of SIBs, the intricacies of the new PyBaMM model, and the exciting possibilities it unlocks for the future of energy storage.
Nov 12, 2024
Run your first virtual battery test today
Simulate, iterate, and validate your cell configurations with no lab time required.
Ionworks Technologies Inc. All rights reserved.
Run your first virtual battery test today
Simulate, iterate, and validate your cell configurations with no lab time required.
Run your first virtual battery test today
Simulate, iterate, and validate your cell configurations with no lab time required.
Ionworks Technologies Inc. All rights reserved.
Run your first virtual battery test today
Simulate, iterate, and validate your cell configurations with no lab time required.
Ionworks Technologies Inc. All rights reserved.