Apr 4, 2025
Apr 4, 2025
How Much Charge Can a Battery Hold? Exploring Battery Capacity
How Much Charge Can a Battery Hold? Exploring Battery Capacity

In the last publication we explored reaction kinetics and how the rate of lithium-ion intercalation impacts battery performance. Now, we turn our focus to a concept at the core of battery performance: battery capacity. Battery capacity quantifies how much charge a battery can store and deliver—making it a critical metric for applications ranging from smartphones to electric vehicles. While the intuitive definition of capacity may seem straightforward, there are multiple ways to measure and report it. In this post, we’ll break down the three main types of capacity: nominal, theoretical, and C-rate-specific.
The first and most common definition of capacity is nominal capacity, which is the value typically provided by the battery’s manufacturer. This is the amount of charge (measured in ampere-hours, Ah) the battery is designed to deliver under standardized test conditions. Nominal capacity serves as a benchmark for comparing different batteries, but it’s important to recognize that it represents an idealized value—hence it’s often reported as a round number. Real-world conditions, such as temperature fluctuations, aging, and faster discharge rates, usually result in a lower usable capacity than the nominal value.
Theoretical capacity (or open-circuit capacity) represents the maximum charge a battery can deliver within a given voltage window (e.g., 4.2 V to 2.5 V) when discharged infinitely slowly. In practice, this means discharging at an extremely low current to eliminate kinetic or transport limitations and to fully utilize the electrodes’ active material. Theoretical capacity is typically calculated from the open-circuit potentials of the electrodes and the total volume of active material. Because it assumes ideal conditions, theoretical capacity always exceeds the capacity measured under practical conditions.
The third way to define capacity involves measuring it at a specific C-rate within a given voltage window. A C-rate indicates how quickly the battery is discharged relative to its nominal capacity. For example, a C-rate of 1C means the battery is discharged in one hour, while a 0.5C rate corresponds to a two-hour discharge. Measuring capacity at a specific C-rate gives a more realistic picture of the battery's performance under actual operating conditions. However, as the C-rate increases, kinetic and transport limitations become significant, leading to lower measured capacities. This definition is particularly important for assessing capacity fade due to degradation. It’s worth noting that the choice of C-rate itself affects the measured capacity, so it’s crucial to account for this when comparing results.
As we’ve seen, battery capacity is far more than just a single number—it’s a nuanced concept that depends heavily on testing methods and conditions. Understanding these different definitions is essential for interpreting battery specifications and modeling battery performance accurately. These nuances also influence a closely related metric: the state of charge (SoC), which provides a real-time snapshot of how much energy remains in a battery. SoC will be the focus of our next post.
In the last publication we explored reaction kinetics and how the rate of lithium-ion intercalation impacts battery performance. Now, we turn our focus to a concept at the core of battery performance: battery capacity. Battery capacity quantifies how much charge a battery can store and deliver—making it a critical metric for applications ranging from smartphones to electric vehicles. While the intuitive definition of capacity may seem straightforward, there are multiple ways to measure and report it. In this post, we’ll break down the three main types of capacity: nominal, theoretical, and C-rate-specific.
The first and most common definition of capacity is nominal capacity, which is the value typically provided by the battery’s manufacturer. This is the amount of charge (measured in ampere-hours, Ah) the battery is designed to deliver under standardized test conditions. Nominal capacity serves as a benchmark for comparing different batteries, but it’s important to recognize that it represents an idealized value—hence it’s often reported as a round number. Real-world conditions, such as temperature fluctuations, aging, and faster discharge rates, usually result in a lower usable capacity than the nominal value.
Theoretical capacity (or open-circuit capacity) represents the maximum charge a battery can deliver within a given voltage window (e.g., 4.2 V to 2.5 V) when discharged infinitely slowly. In practice, this means discharging at an extremely low current to eliminate kinetic or transport limitations and to fully utilize the electrodes’ active material. Theoretical capacity is typically calculated from the open-circuit potentials of the electrodes and the total volume of active material. Because it assumes ideal conditions, theoretical capacity always exceeds the capacity measured under practical conditions.
The third way to define capacity involves measuring it at a specific C-rate within a given voltage window. A C-rate indicates how quickly the battery is discharged relative to its nominal capacity. For example, a C-rate of 1C means the battery is discharged in one hour, while a 0.5C rate corresponds to a two-hour discharge. Measuring capacity at a specific C-rate gives a more realistic picture of the battery's performance under actual operating conditions. However, as the C-rate increases, kinetic and transport limitations become significant, leading to lower measured capacities. This definition is particularly important for assessing capacity fade due to degradation. It’s worth noting that the choice of C-rate itself affects the measured capacity, so it’s crucial to account for this when comparing results.
As we’ve seen, battery capacity is far more than just a single number—it’s a nuanced concept that depends heavily on testing methods and conditions. Understanding these different definitions is essential for interpreting battery specifications and modeling battery performance accurately. These nuances also influence a closely related metric: the state of charge (SoC), which provides a real-time snapshot of how much energy remains in a battery. SoC will be the focus of our next post.
In the last publication we explored reaction kinetics and how the rate of lithium-ion intercalation impacts battery performance. Now, we turn our focus to a concept at the core of battery performance: battery capacity. Battery capacity quantifies how much charge a battery can store and deliver—making it a critical metric for applications ranging from smartphones to electric vehicles. While the intuitive definition of capacity may seem straightforward, there are multiple ways to measure and report it. In this post, we’ll break down the three main types of capacity: nominal, theoretical, and C-rate-specific.
The first and most common definition of capacity is nominal capacity, which is the value typically provided by the battery’s manufacturer. This is the amount of charge (measured in ampere-hours, Ah) the battery is designed to deliver under standardized test conditions. Nominal capacity serves as a benchmark for comparing different batteries, but it’s important to recognize that it represents an idealized value—hence it’s often reported as a round number. Real-world conditions, such as temperature fluctuations, aging, and faster discharge rates, usually result in a lower usable capacity than the nominal value.
Theoretical capacity (or open-circuit capacity) represents the maximum charge a battery can deliver within a given voltage window (e.g., 4.2 V to 2.5 V) when discharged infinitely slowly. In practice, this means discharging at an extremely low current to eliminate kinetic or transport limitations and to fully utilize the electrodes’ active material. Theoretical capacity is typically calculated from the open-circuit potentials of the electrodes and the total volume of active material. Because it assumes ideal conditions, theoretical capacity always exceeds the capacity measured under practical conditions.
The third way to define capacity involves measuring it at a specific C-rate within a given voltage window. A C-rate indicates how quickly the battery is discharged relative to its nominal capacity. For example, a C-rate of 1C means the battery is discharged in one hour, while a 0.5C rate corresponds to a two-hour discharge. Measuring capacity at a specific C-rate gives a more realistic picture of the battery's performance under actual operating conditions. However, as the C-rate increases, kinetic and transport limitations become significant, leading to lower measured capacities. This definition is particularly important for assessing capacity fade due to degradation. It’s worth noting that the choice of C-rate itself affects the measured capacity, so it’s crucial to account for this when comparing results.
As we’ve seen, battery capacity is far more than just a single number—it’s a nuanced concept that depends heavily on testing methods and conditions. Understanding these different definitions is essential for interpreting battery specifications and modeling battery performance accurately. These nuances also influence a closely related metric: the state of charge (SoC), which provides a real-time snapshot of how much energy remains in a battery. SoC will be the focus of our next post.
In the last publication we explored reaction kinetics and how the rate of lithium-ion intercalation impacts battery performance. Now, we turn our focus to a concept at the core of battery performance: battery capacity. Battery capacity quantifies how much charge a battery can store and deliver—making it a critical metric for applications ranging from smartphones to electric vehicles. While the intuitive definition of capacity may seem straightforward, there are multiple ways to measure and report it. In this post, we’ll break down the three main types of capacity: nominal, theoretical, and C-rate-specific.
The first and most common definition of capacity is nominal capacity, which is the value typically provided by the battery’s manufacturer. This is the amount of charge (measured in ampere-hours, Ah) the battery is designed to deliver under standardized test conditions. Nominal capacity serves as a benchmark for comparing different batteries, but it’s important to recognize that it represents an idealized value—hence it’s often reported as a round number. Real-world conditions, such as temperature fluctuations, aging, and faster discharge rates, usually result in a lower usable capacity than the nominal value.
Theoretical capacity (or open-circuit capacity) represents the maximum charge a battery can deliver within a given voltage window (e.g., 4.2 V to 2.5 V) when discharged infinitely slowly. In practice, this means discharging at an extremely low current to eliminate kinetic or transport limitations and to fully utilize the electrodes’ active material. Theoretical capacity is typically calculated from the open-circuit potentials of the electrodes and the total volume of active material. Because it assumes ideal conditions, theoretical capacity always exceeds the capacity measured under practical conditions.
The third way to define capacity involves measuring it at a specific C-rate within a given voltage window. A C-rate indicates how quickly the battery is discharged relative to its nominal capacity. For example, a C-rate of 1C means the battery is discharged in one hour, while a 0.5C rate corresponds to a two-hour discharge. Measuring capacity at a specific C-rate gives a more realistic picture of the battery's performance under actual operating conditions. However, as the C-rate increases, kinetic and transport limitations become significant, leading to lower measured capacities. This definition is particularly important for assessing capacity fade due to degradation. It’s worth noting that the choice of C-rate itself affects the measured capacity, so it’s crucial to account for this when comparing results.
As we’ve seen, battery capacity is far more than just a single number—it’s a nuanced concept that depends heavily on testing methods and conditions. Understanding these different definitions is essential for interpreting battery specifications and modeling battery performance accurately. These nuances also influence a closely related metric: the state of charge (SoC), which provides a real-time snapshot of how much energy remains in a battery. SoC will be the focus of our next post.
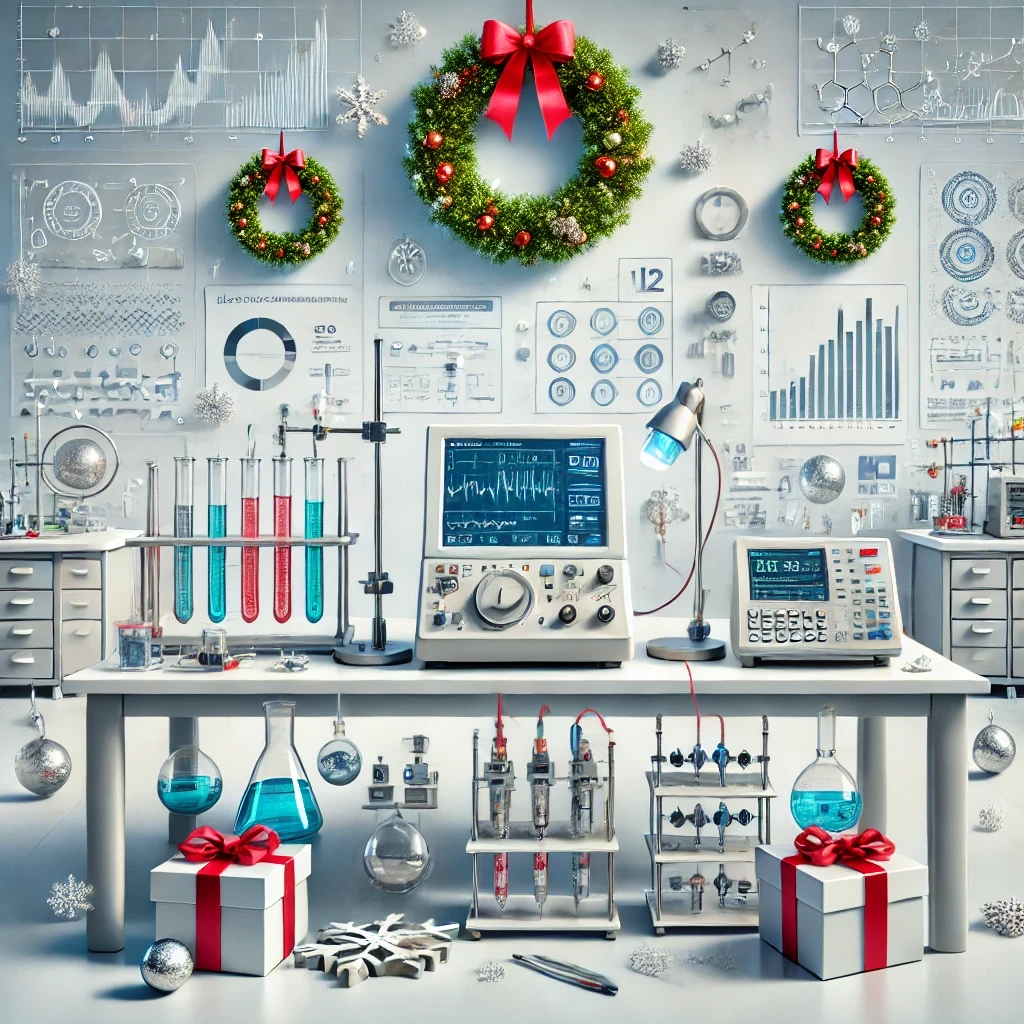
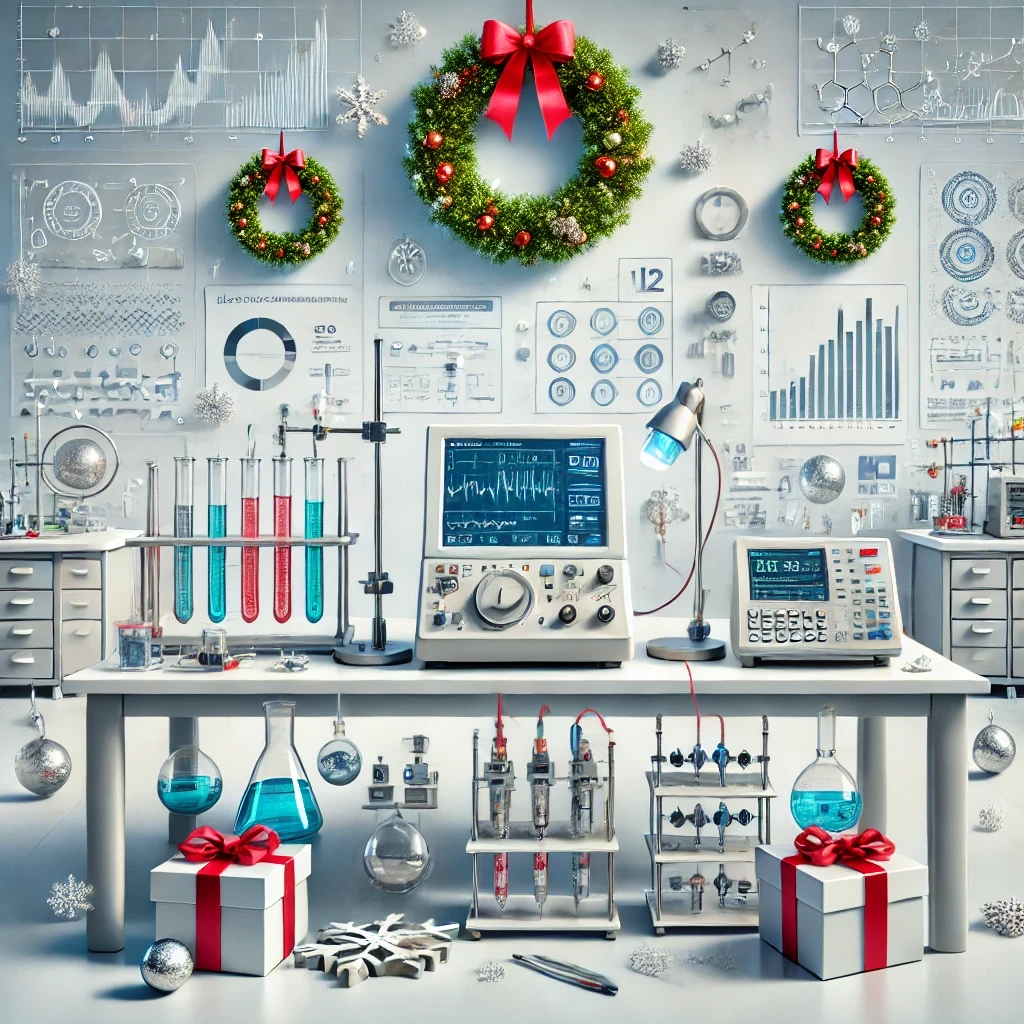
12 Days of Electrochemical Testing
To celebrate the holiday season and the re-release of Ionworks Studio, we featured "12 (business) days of electrochemical testing". Each day we pick a test, give a little bit of information about it, and show you how to run it in Ionworks. 🔋 🎄
Dec 17, 2024
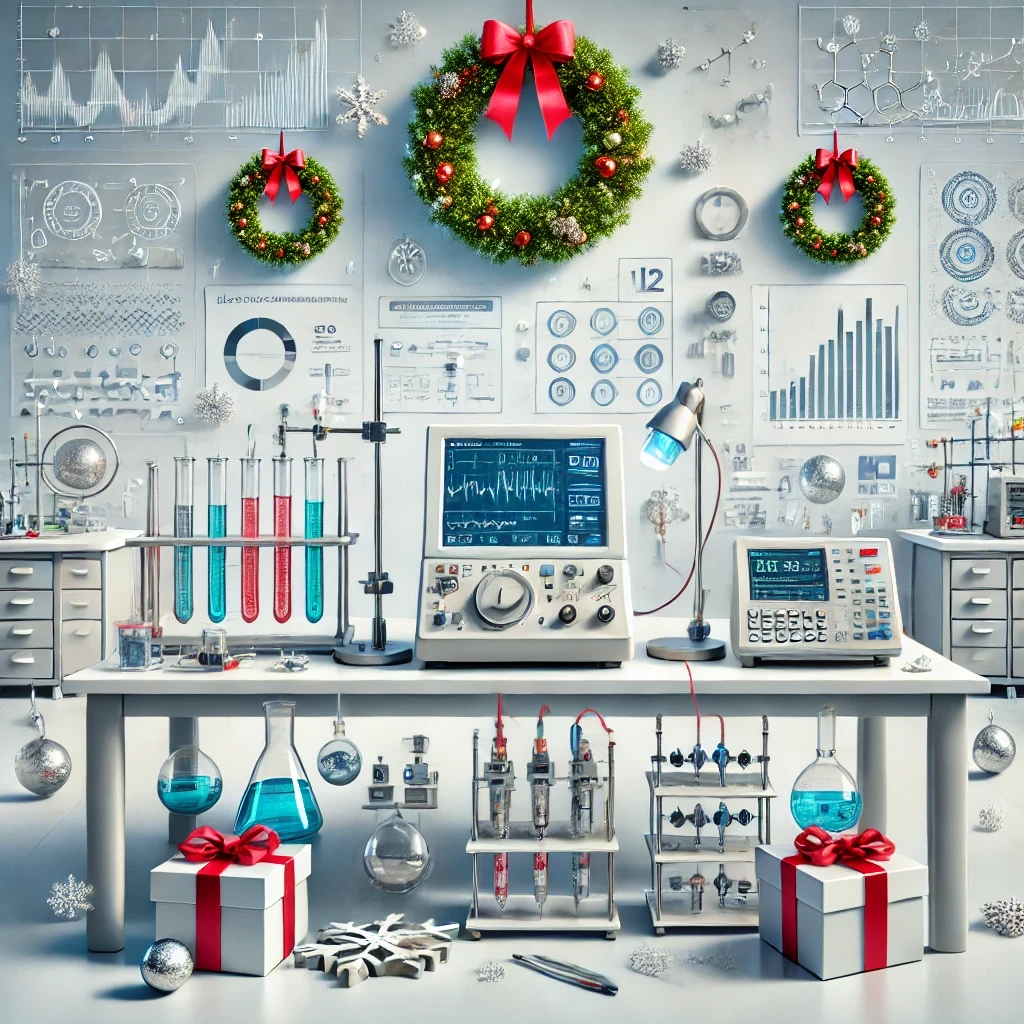
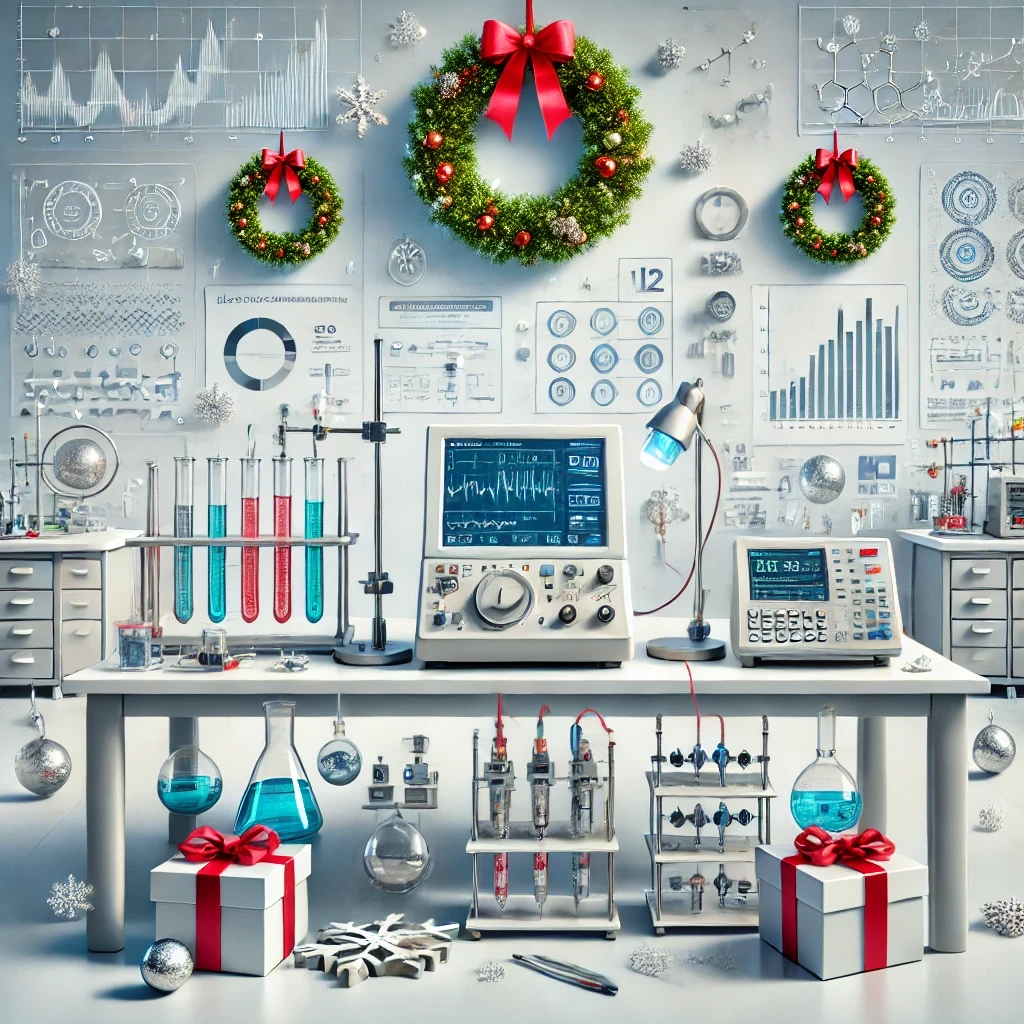
12 Days of Electrochemical Testing
To celebrate the holiday season and the re-release of Ionworks Studio, we featured "12 (business) days of electrochemical testing". Each day we pick a test, give a little bit of information about it, and show you how to run it in Ionworks. 🔋 🎄
Dec 17, 2024
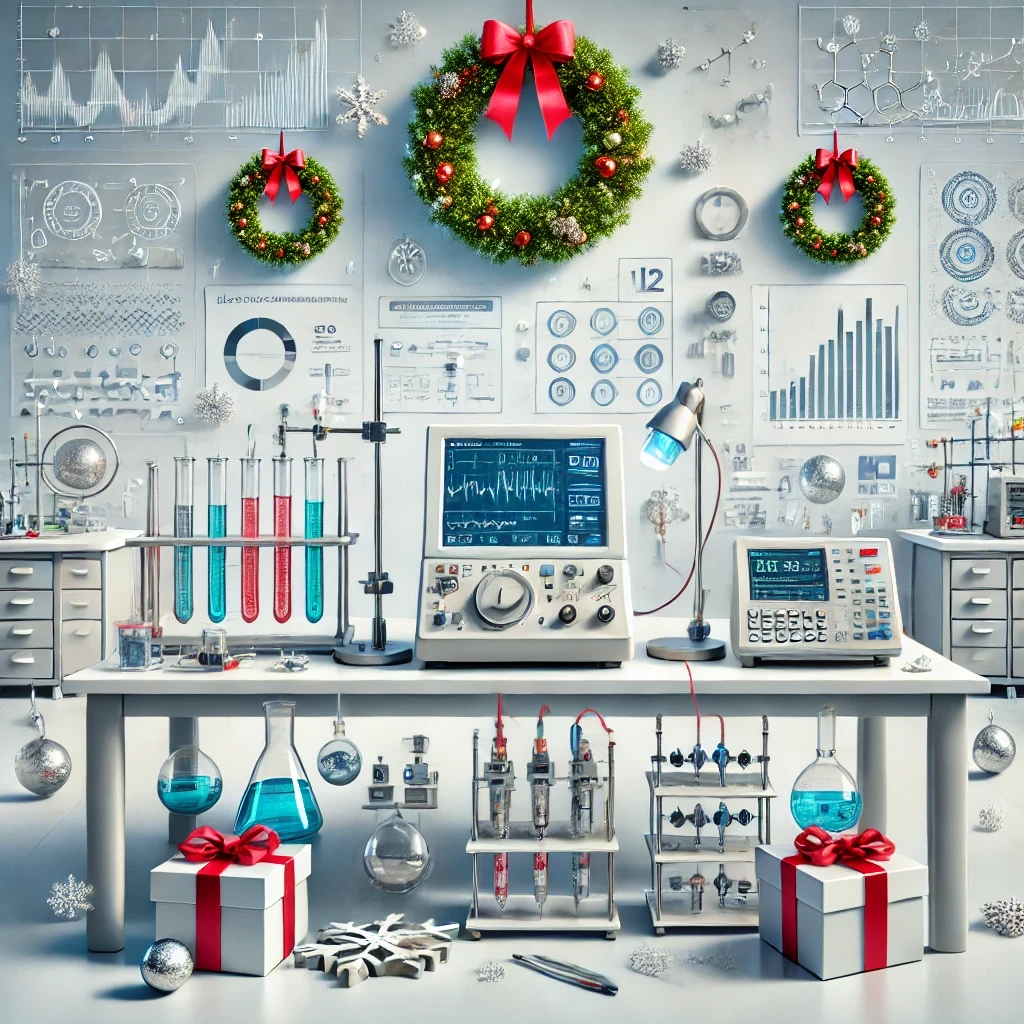
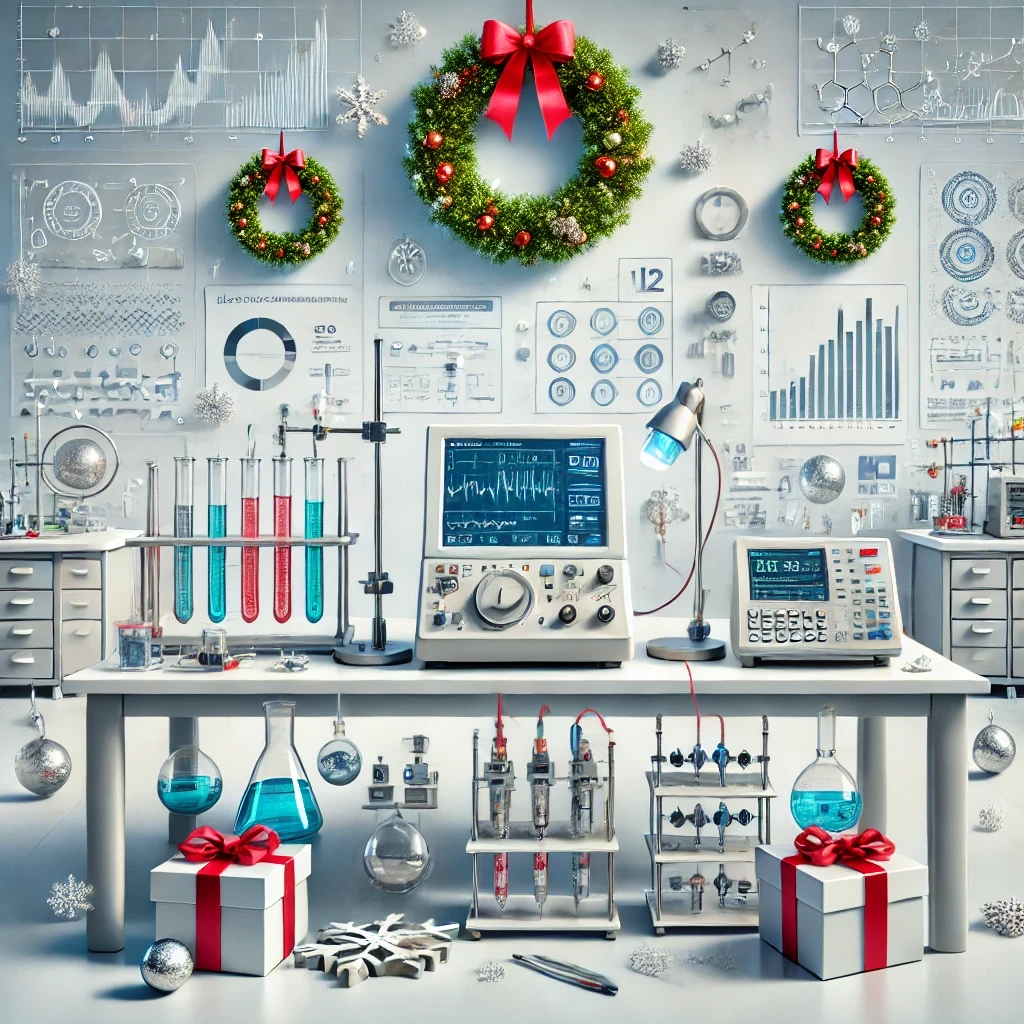
12 Days of Electrochemical Testing
To celebrate the holiday season and the re-release of Ionworks Studio, we featured "12 (business) days of electrochemical testing". Each day we pick a test, give a little bit of information about it, and show you how to run it in Ionworks. 🔋 🎄
Dec 17, 2024
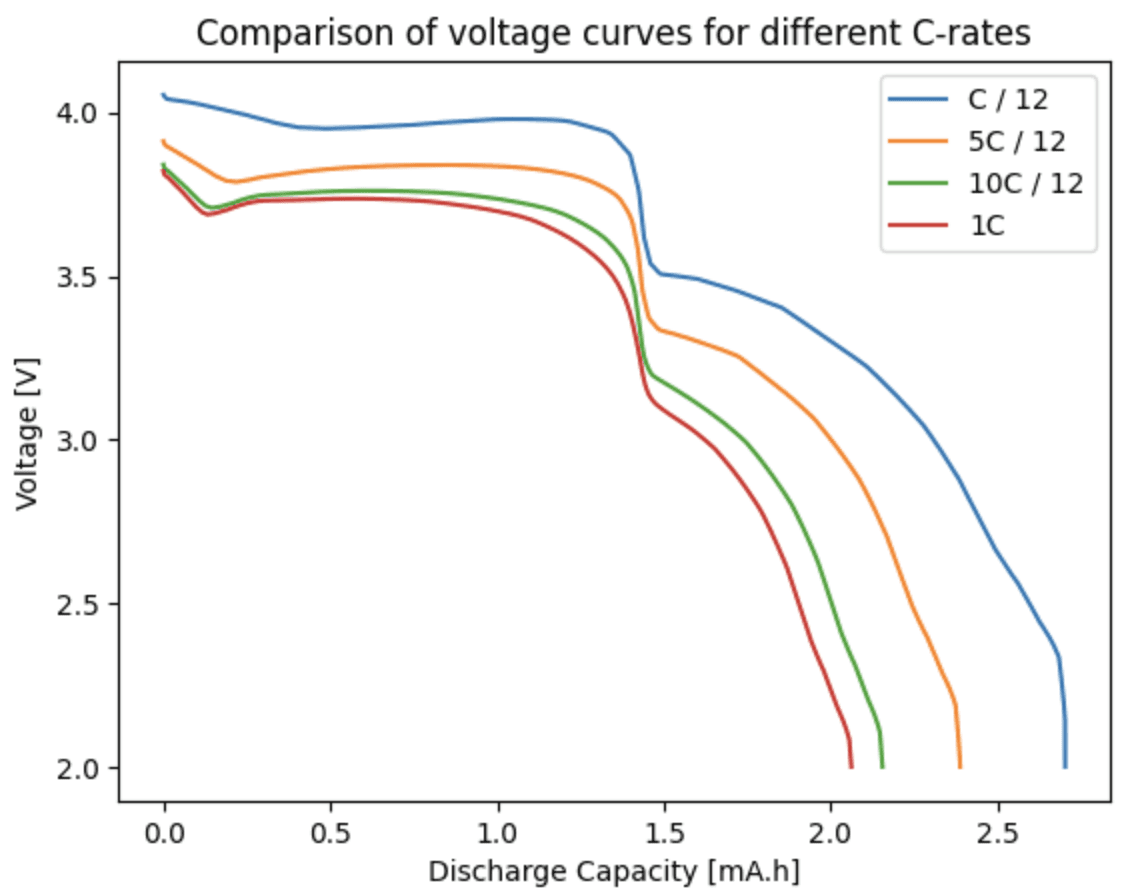
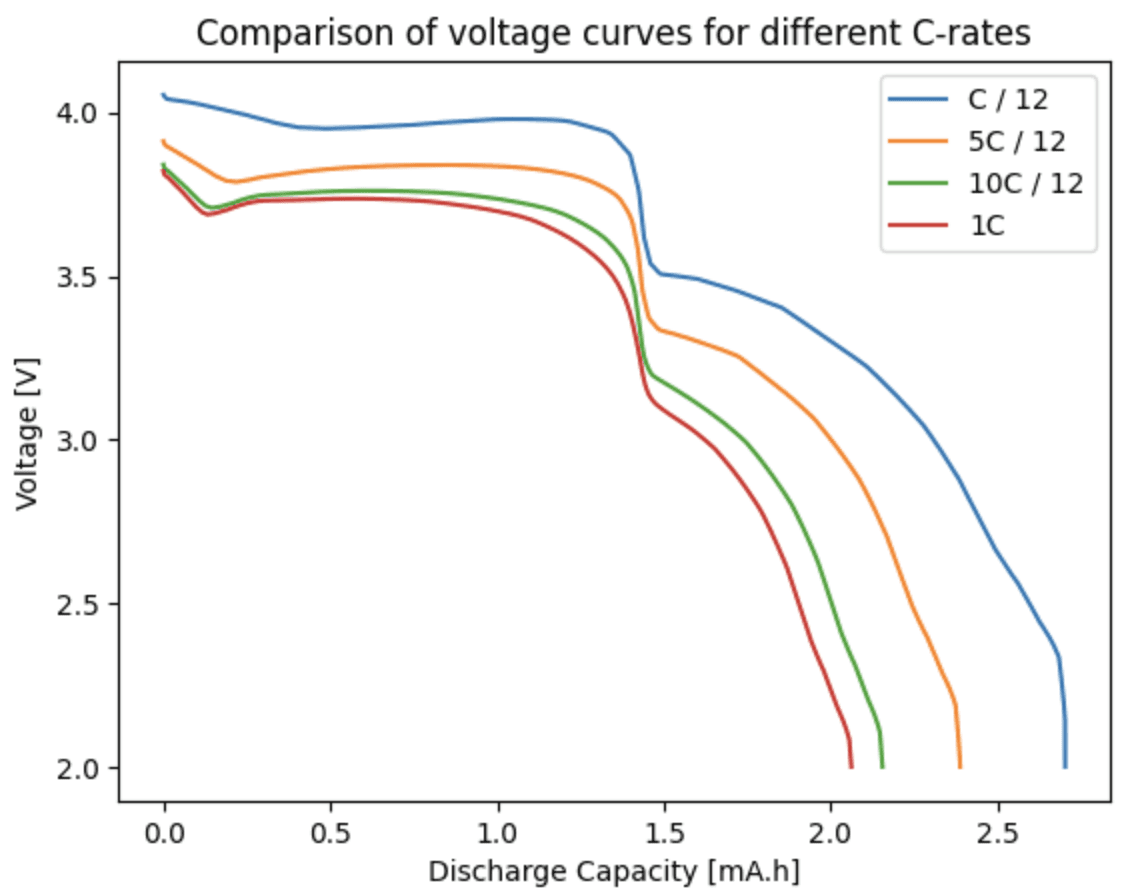
Sodium Ion Battery Model now available in PyBaMM!
This blog post explores the history of SIBs, the intricacies of the new PyBaMM model, and the exciting possibilities it unlocks for the future of energy storage.
Nov 12, 2024
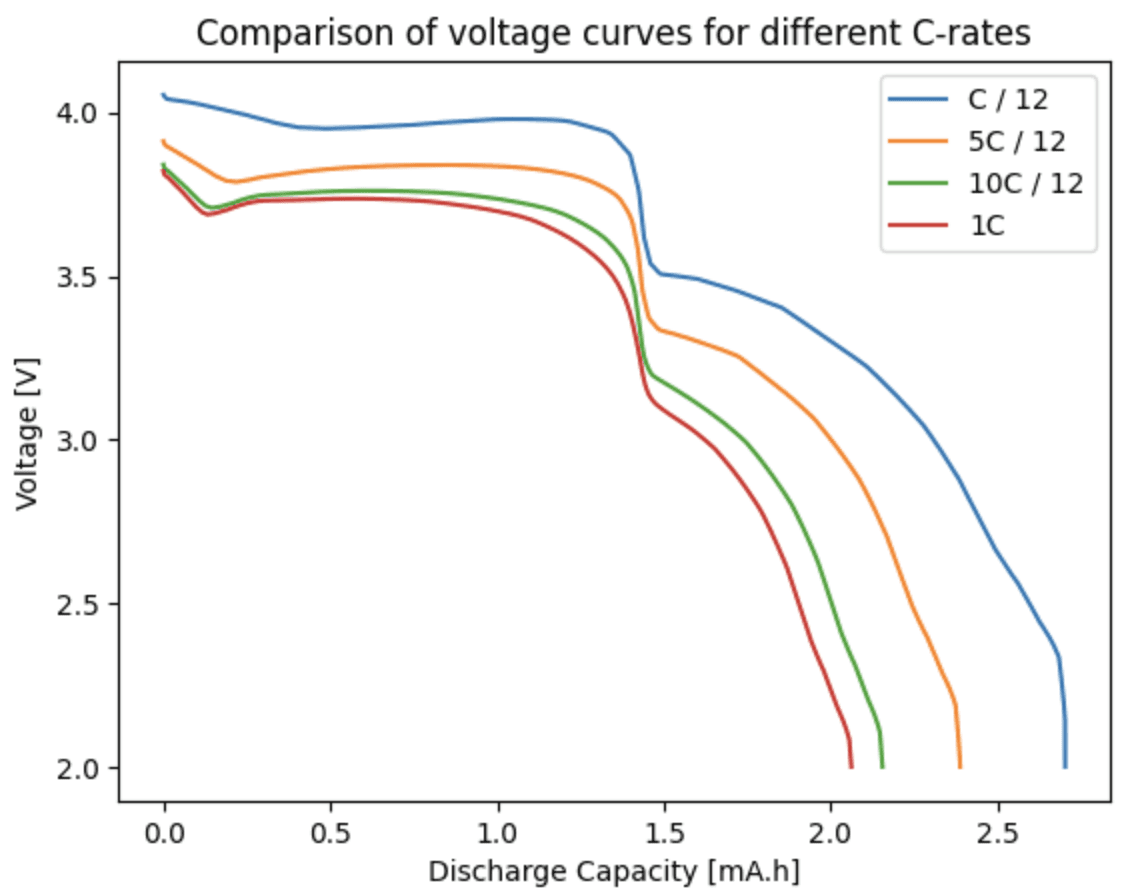
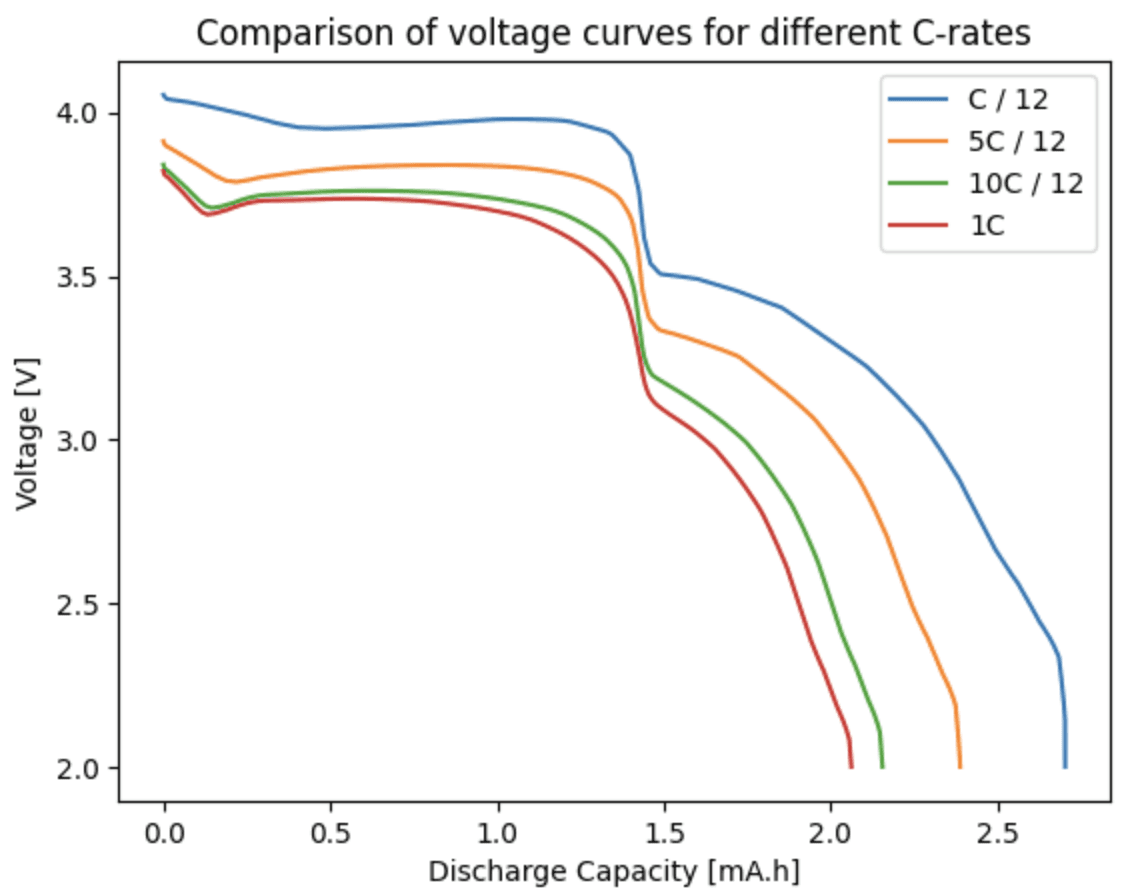
Sodium Ion Battery Model now available in PyBaMM!
This blog post explores the history of SIBs, the intricacies of the new PyBaMM model, and the exciting possibilities it unlocks for the future of energy storage.
Nov 12, 2024
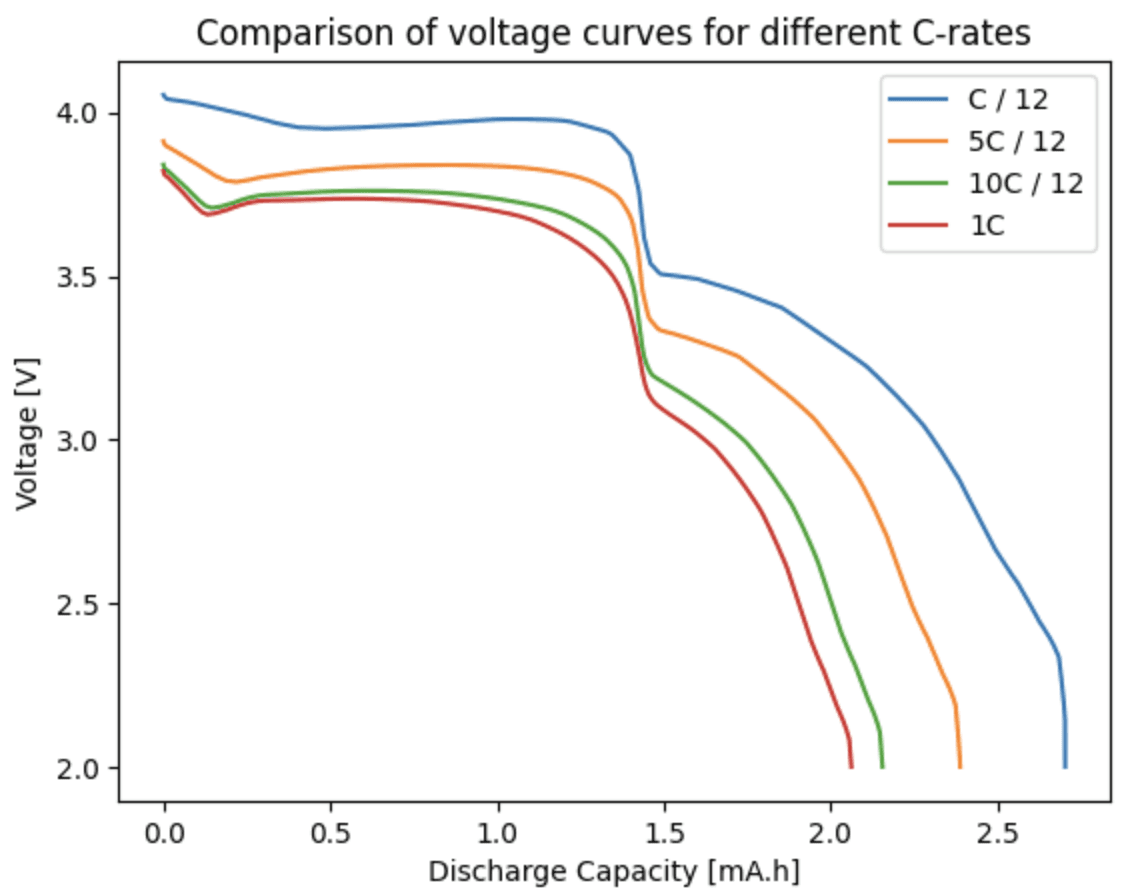
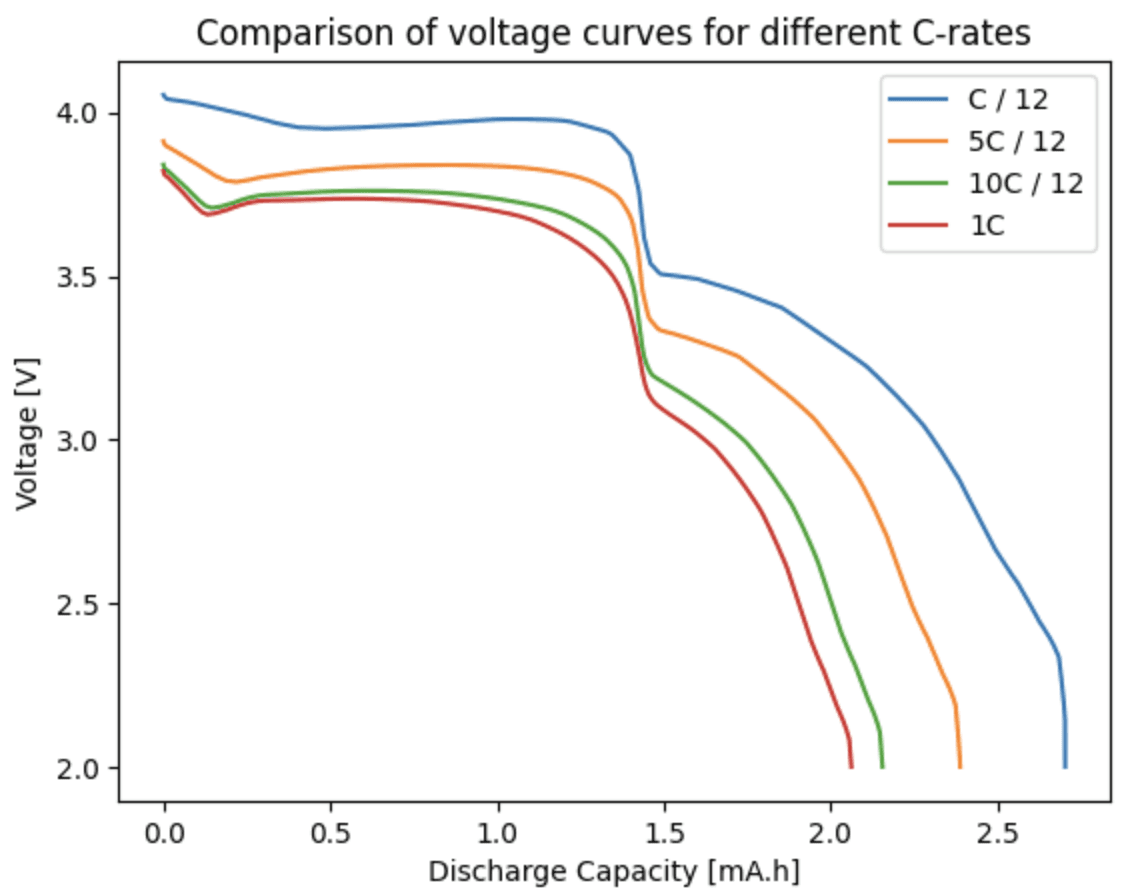
Sodium Ion Battery Model now available in PyBaMM!
This blog post explores the history of SIBs, the intricacies of the new PyBaMM model, and the exciting possibilities it unlocks for the future of energy storage.
Nov 12, 2024
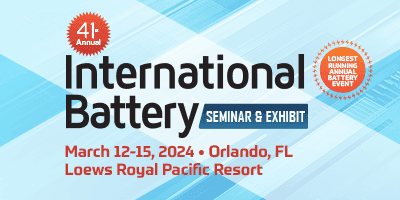
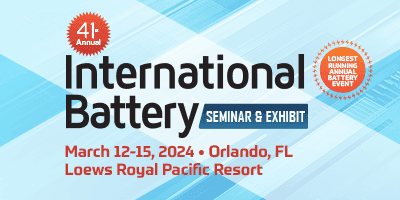
Ionworks Presents at International Battery Seminar
Ionworks CEO Valentin Sulzer presents at the International Battery Seminar in Florida
Mar 12, 2024
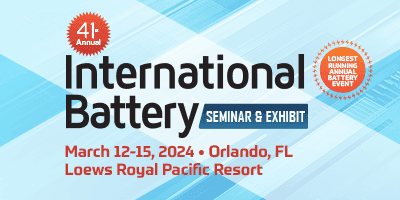
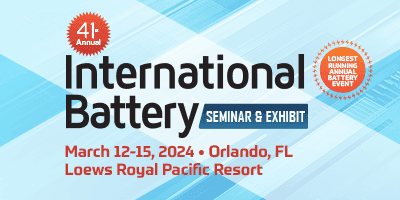
Ionworks Presents at International Battery Seminar
Ionworks CEO Valentin Sulzer presents at the International Battery Seminar in Florida
Mar 12, 2024
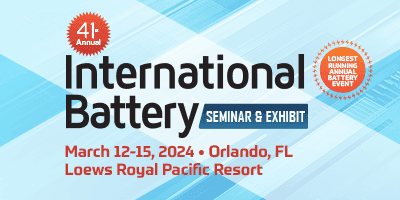
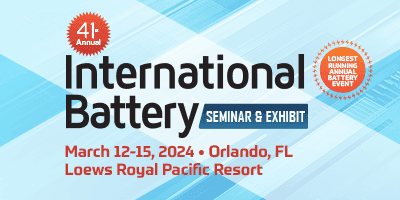
Ionworks Presents at International Battery Seminar
Ionworks CEO Valentin Sulzer presents at the International Battery Seminar in Florida
Mar 12, 2024
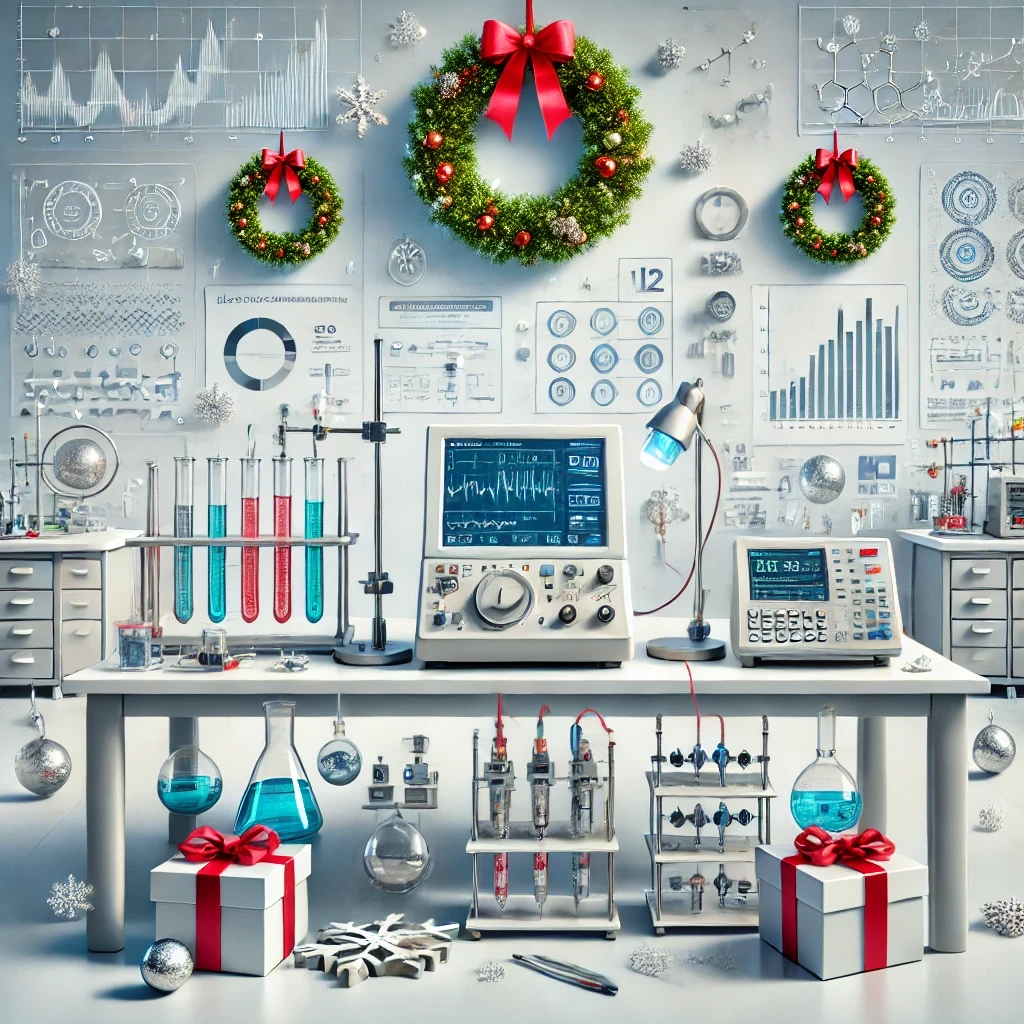
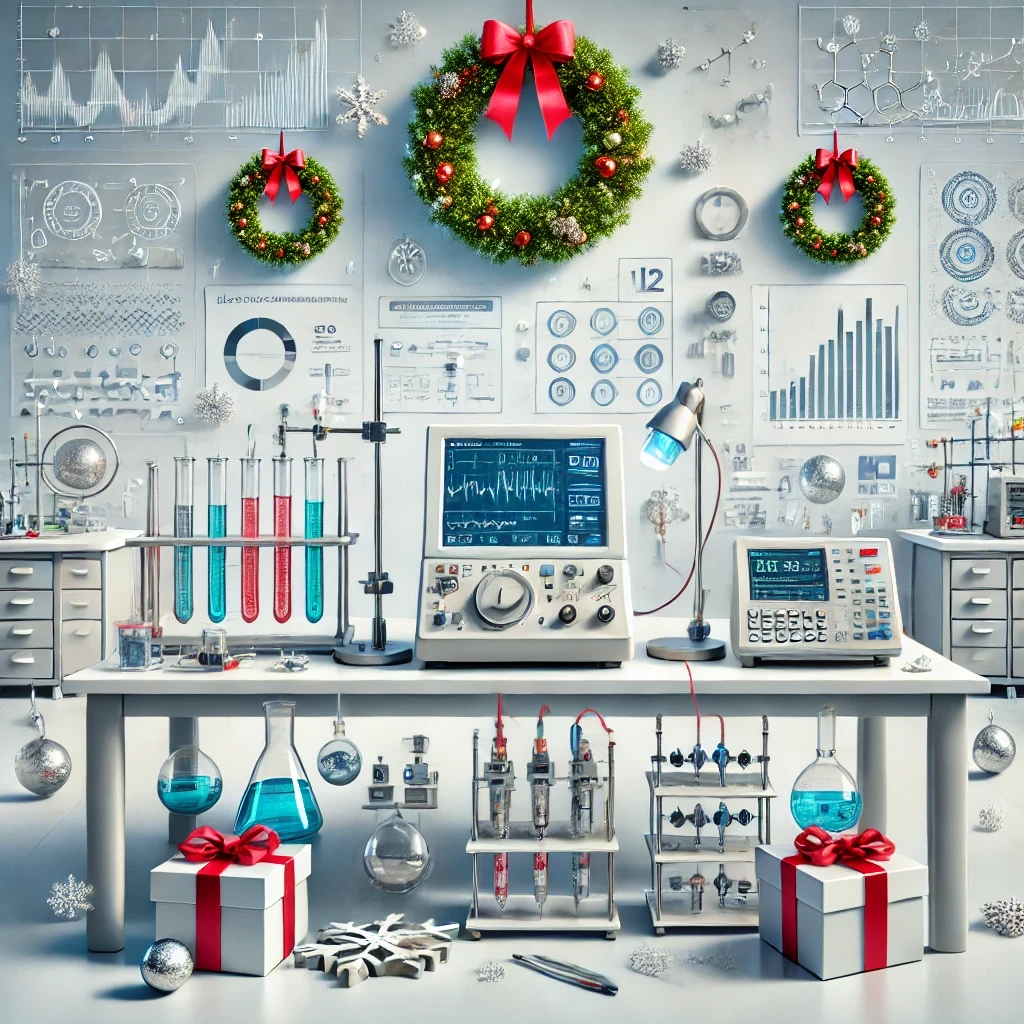
12 Days of Electrochemical Testing
To celebrate the holiday season and the re-release of Ionworks Studio, we featured "12 (business) days of electrochemical testing". Each day we pick a test, give a little bit of information about it, and show you how to run it in Ionworks. 🔋 🎄
Dec 17, 2024
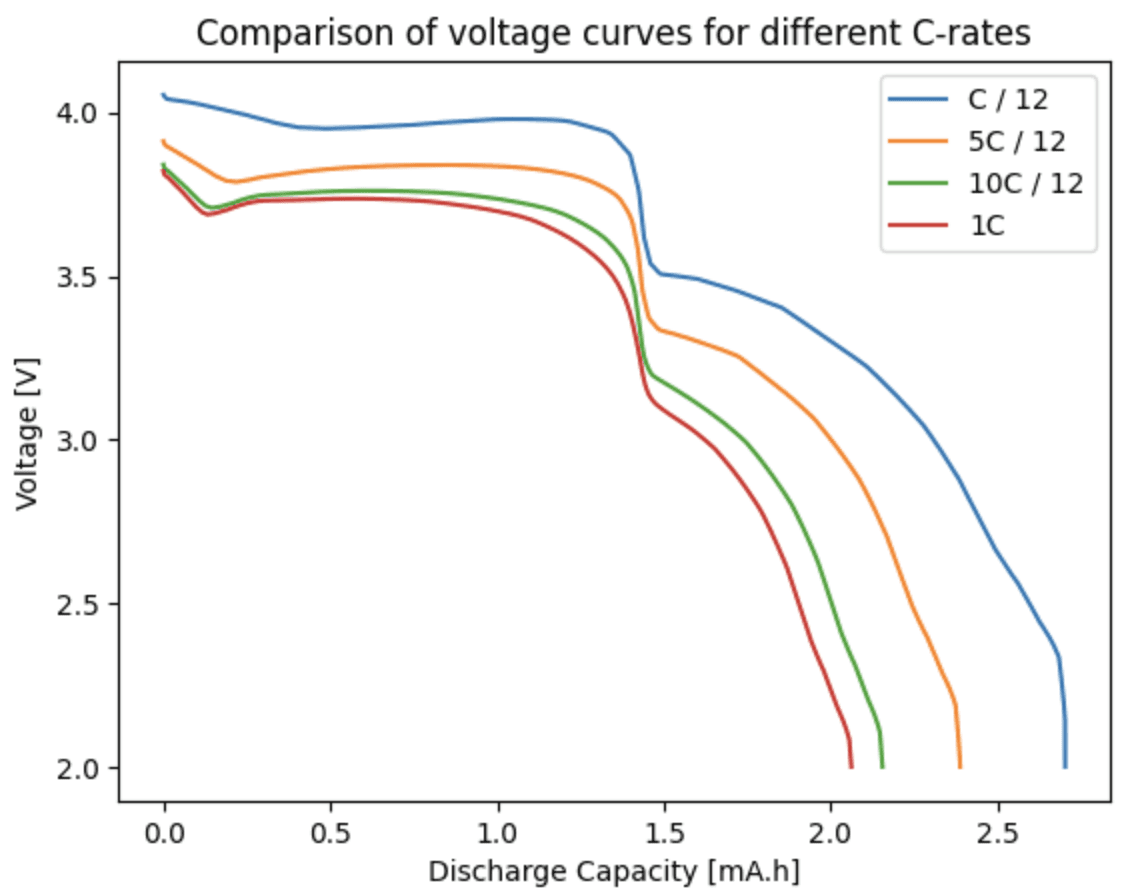
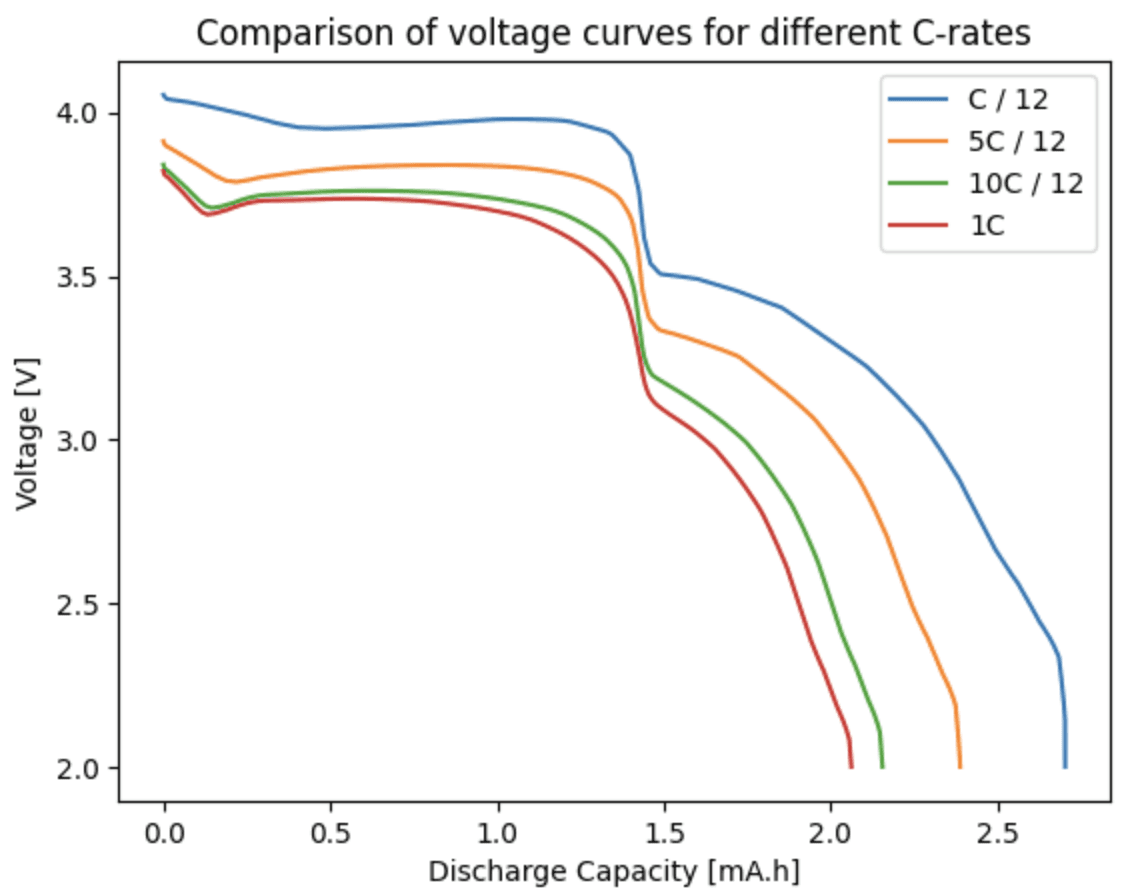
Sodium Ion Battery Model now available in PyBaMM!
This blog post explores the history of SIBs, the intricacies of the new PyBaMM model, and the exciting possibilities it unlocks for the future of energy storage.
Nov 12, 2024
Run your first virtual battery test today
Simulate, iterate, and validate your cell configurations with no lab time required.
Ionworks Technologies Inc. All rights reserved.
Run your first virtual battery test today
Simulate, iterate, and validate your cell configurations with no lab time required.
Run your first virtual battery test today
Simulate, iterate, and validate your cell configurations with no lab time required.
Ionworks Technologies Inc. All rights reserved.
Run your first virtual battery test today
Simulate, iterate, and validate your cell configurations with no lab time required.
Ionworks Technologies Inc. All rights reserved.