Apr 5, 2025
Apr 5, 2025
How Full is Your Battery? Defining the State of Charge (SoC)
How Full is Your Battery? Defining the State of Charge (SoC)

Previously, we explored the concept of battery capacity and discussed the various ways it can be measured. However, knowing how much capacity a battery can store is only part of the picture. To use a battery effectively, we also need to understand how much capacity is currently available. This brings us to the concept of State of Charge (SoC)—a dynamic measure of how much charge remains in a battery at any given moment, relative to its total capacity. SoC is an essential parameter for estimating runtime, managing energy, and protecting batteries from overcharging or over-discharging.
State of Charge (SoC) is expressed as a percentage: 100% represents a fully charged battery, while 0% indicates the battery is empty. While this definition may seem intuitive, the details of accurately determining SoC can be complex. Two primary methods for estimating SoC are Coulomb counting and voltage-based measurement.
Coulomb counting, sometimes called current integration, is one of the most widely used methods for SoC estimation. This approach calculates SoC by measuring the current flowing into or out of the battery over time. Since current represents the rate of charge flow, integrating it over time gives the total charge transferred. Starting from a known initial SoC and the battery’s total capacity, the updated SoC can be determined. While precise over short periods, this method is prone to cumulative errors over time due to factors like measurement inaccuracies and side reactions (e.g., self-discharge).
Open-circuit voltage (OCV) is another commonly used approach to estimating SoC. As we discussed in our post on open-circuit potentials, the OCV of a battery correlates directly with its SoC. Many manufacturers provide OCV-SoC curves that map the relationship between voltage and SoC for a specific battery chemistry. By measuring the battery’s OCV after it has rested (with no current flowing), the SoC can be estimated accurately.
In some cases, the actual voltage of a battery under load is used to estimate SoC. However, this requires caution. While OCV has a well-defined relationship with SoC, the voltage measured during operation also reflects transient effects such as Ohmic losses and overpotentials. One example is polarization: after a discharge, the voltage often rises slightly as lithium concentrations equilibrate in the electrodes and electrolyte. If this operational voltage is used naively to estimate SoC, it may appear that the SoC increases after discharge stops—an incorrect result since no additional charge has entered the battery.
It’s also important to consider how battery aging affects SoC estimation. As a battery ages, its total capacity decreases, which can cause a mismatch between the estimated and actual SoC if capacity fade is not accounted for. Advanced battery management systems (BMS) dynamically adjust the total capacity over time, improving SoC accuracy throughout the battery’s lifespan.
State of Charge, together with capacity, is vital for monitoring and managing battery performance, but these metrics are not the full story. In our next post, we’ll explore another key factor: internal resistance, which plays a critical role in efficiency, heat generation, and power output.
Previously, we explored the concept of battery capacity and discussed the various ways it can be measured. However, knowing how much capacity a battery can store is only part of the picture. To use a battery effectively, we also need to understand how much capacity is currently available. This brings us to the concept of State of Charge (SoC)—a dynamic measure of how much charge remains in a battery at any given moment, relative to its total capacity. SoC is an essential parameter for estimating runtime, managing energy, and protecting batteries from overcharging or over-discharging.
State of Charge (SoC) is expressed as a percentage: 100% represents a fully charged battery, while 0% indicates the battery is empty. While this definition may seem intuitive, the details of accurately determining SoC can be complex. Two primary methods for estimating SoC are Coulomb counting and voltage-based measurement.
Coulomb counting, sometimes called current integration, is one of the most widely used methods for SoC estimation. This approach calculates SoC by measuring the current flowing into or out of the battery over time. Since current represents the rate of charge flow, integrating it over time gives the total charge transferred. Starting from a known initial SoC and the battery’s total capacity, the updated SoC can be determined. While precise over short periods, this method is prone to cumulative errors over time due to factors like measurement inaccuracies and side reactions (e.g., self-discharge).
Open-circuit voltage (OCV) is another commonly used approach to estimating SoC. As we discussed in our post on open-circuit potentials, the OCV of a battery correlates directly with its SoC. Many manufacturers provide OCV-SoC curves that map the relationship between voltage and SoC for a specific battery chemistry. By measuring the battery’s OCV after it has rested (with no current flowing), the SoC can be estimated accurately.
In some cases, the actual voltage of a battery under load is used to estimate SoC. However, this requires caution. While OCV has a well-defined relationship with SoC, the voltage measured during operation also reflects transient effects such as Ohmic losses and overpotentials. One example is polarization: after a discharge, the voltage often rises slightly as lithium concentrations equilibrate in the electrodes and electrolyte. If this operational voltage is used naively to estimate SoC, it may appear that the SoC increases after discharge stops—an incorrect result since no additional charge has entered the battery.
It’s also important to consider how battery aging affects SoC estimation. As a battery ages, its total capacity decreases, which can cause a mismatch between the estimated and actual SoC if capacity fade is not accounted for. Advanced battery management systems (BMS) dynamically adjust the total capacity over time, improving SoC accuracy throughout the battery’s lifespan.
State of Charge, together with capacity, is vital for monitoring and managing battery performance, but these metrics are not the full story. In our next post, we’ll explore another key factor: internal resistance, which plays a critical role in efficiency, heat generation, and power output.
Previously, we explored the concept of battery capacity and discussed the various ways it can be measured. However, knowing how much capacity a battery can store is only part of the picture. To use a battery effectively, we also need to understand how much capacity is currently available. This brings us to the concept of State of Charge (SoC)—a dynamic measure of how much charge remains in a battery at any given moment, relative to its total capacity. SoC is an essential parameter for estimating runtime, managing energy, and protecting batteries from overcharging or over-discharging.
State of Charge (SoC) is expressed as a percentage: 100% represents a fully charged battery, while 0% indicates the battery is empty. While this definition may seem intuitive, the details of accurately determining SoC can be complex. Two primary methods for estimating SoC are Coulomb counting and voltage-based measurement.
Coulomb counting, sometimes called current integration, is one of the most widely used methods for SoC estimation. This approach calculates SoC by measuring the current flowing into or out of the battery over time. Since current represents the rate of charge flow, integrating it over time gives the total charge transferred. Starting from a known initial SoC and the battery’s total capacity, the updated SoC can be determined. While precise over short periods, this method is prone to cumulative errors over time due to factors like measurement inaccuracies and side reactions (e.g., self-discharge).
Open-circuit voltage (OCV) is another commonly used approach to estimating SoC. As we discussed in our post on open-circuit potentials, the OCV of a battery correlates directly with its SoC. Many manufacturers provide OCV-SoC curves that map the relationship between voltage and SoC for a specific battery chemistry. By measuring the battery’s OCV after it has rested (with no current flowing), the SoC can be estimated accurately.
In some cases, the actual voltage of a battery under load is used to estimate SoC. However, this requires caution. While OCV has a well-defined relationship with SoC, the voltage measured during operation also reflects transient effects such as Ohmic losses and overpotentials. One example is polarization: after a discharge, the voltage often rises slightly as lithium concentrations equilibrate in the electrodes and electrolyte. If this operational voltage is used naively to estimate SoC, it may appear that the SoC increases after discharge stops—an incorrect result since no additional charge has entered the battery.
It’s also important to consider how battery aging affects SoC estimation. As a battery ages, its total capacity decreases, which can cause a mismatch between the estimated and actual SoC if capacity fade is not accounted for. Advanced battery management systems (BMS) dynamically adjust the total capacity over time, improving SoC accuracy throughout the battery’s lifespan.
State of Charge, together with capacity, is vital for monitoring and managing battery performance, but these metrics are not the full story. In our next post, we’ll explore another key factor: internal resistance, which plays a critical role in efficiency, heat generation, and power output.
Previously, we explored the concept of battery capacity and discussed the various ways it can be measured. However, knowing how much capacity a battery can store is only part of the picture. To use a battery effectively, we also need to understand how much capacity is currently available. This brings us to the concept of State of Charge (SoC)—a dynamic measure of how much charge remains in a battery at any given moment, relative to its total capacity. SoC is an essential parameter for estimating runtime, managing energy, and protecting batteries from overcharging or over-discharging.
State of Charge (SoC) is expressed as a percentage: 100% represents a fully charged battery, while 0% indicates the battery is empty. While this definition may seem intuitive, the details of accurately determining SoC can be complex. Two primary methods for estimating SoC are Coulomb counting and voltage-based measurement.
Coulomb counting, sometimes called current integration, is one of the most widely used methods for SoC estimation. This approach calculates SoC by measuring the current flowing into or out of the battery over time. Since current represents the rate of charge flow, integrating it over time gives the total charge transferred. Starting from a known initial SoC and the battery’s total capacity, the updated SoC can be determined. While precise over short periods, this method is prone to cumulative errors over time due to factors like measurement inaccuracies and side reactions (e.g., self-discharge).
Open-circuit voltage (OCV) is another commonly used approach to estimating SoC. As we discussed in our post on open-circuit potentials, the OCV of a battery correlates directly with its SoC. Many manufacturers provide OCV-SoC curves that map the relationship between voltage and SoC for a specific battery chemistry. By measuring the battery’s OCV after it has rested (with no current flowing), the SoC can be estimated accurately.
In some cases, the actual voltage of a battery under load is used to estimate SoC. However, this requires caution. While OCV has a well-defined relationship with SoC, the voltage measured during operation also reflects transient effects such as Ohmic losses and overpotentials. One example is polarization: after a discharge, the voltage often rises slightly as lithium concentrations equilibrate in the electrodes and electrolyte. If this operational voltage is used naively to estimate SoC, it may appear that the SoC increases after discharge stops—an incorrect result since no additional charge has entered the battery.
It’s also important to consider how battery aging affects SoC estimation. As a battery ages, its total capacity decreases, which can cause a mismatch between the estimated and actual SoC if capacity fade is not accounted for. Advanced battery management systems (BMS) dynamically adjust the total capacity over time, improving SoC accuracy throughout the battery’s lifespan.
State of Charge, together with capacity, is vital for monitoring and managing battery performance, but these metrics are not the full story. In our next post, we’ll explore another key factor: internal resistance, which plays a critical role in efficiency, heat generation, and power output.
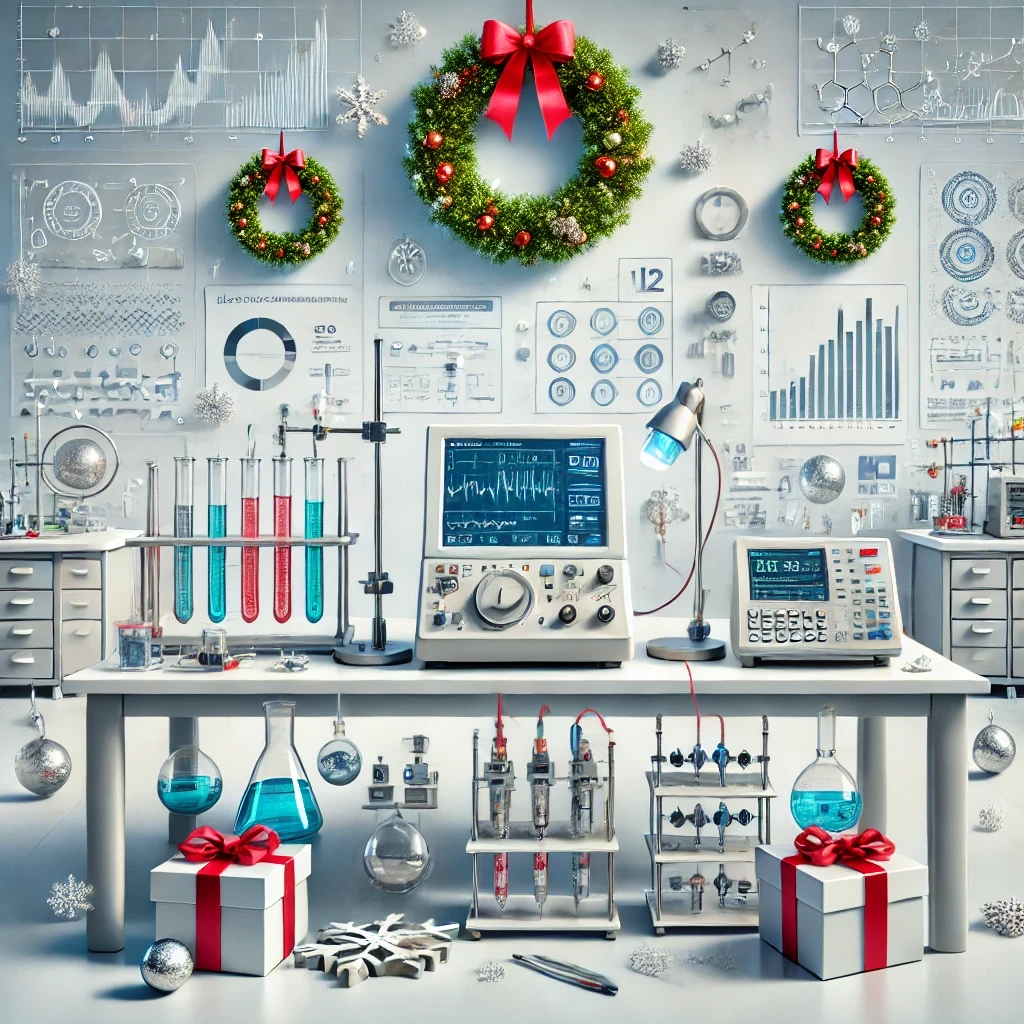
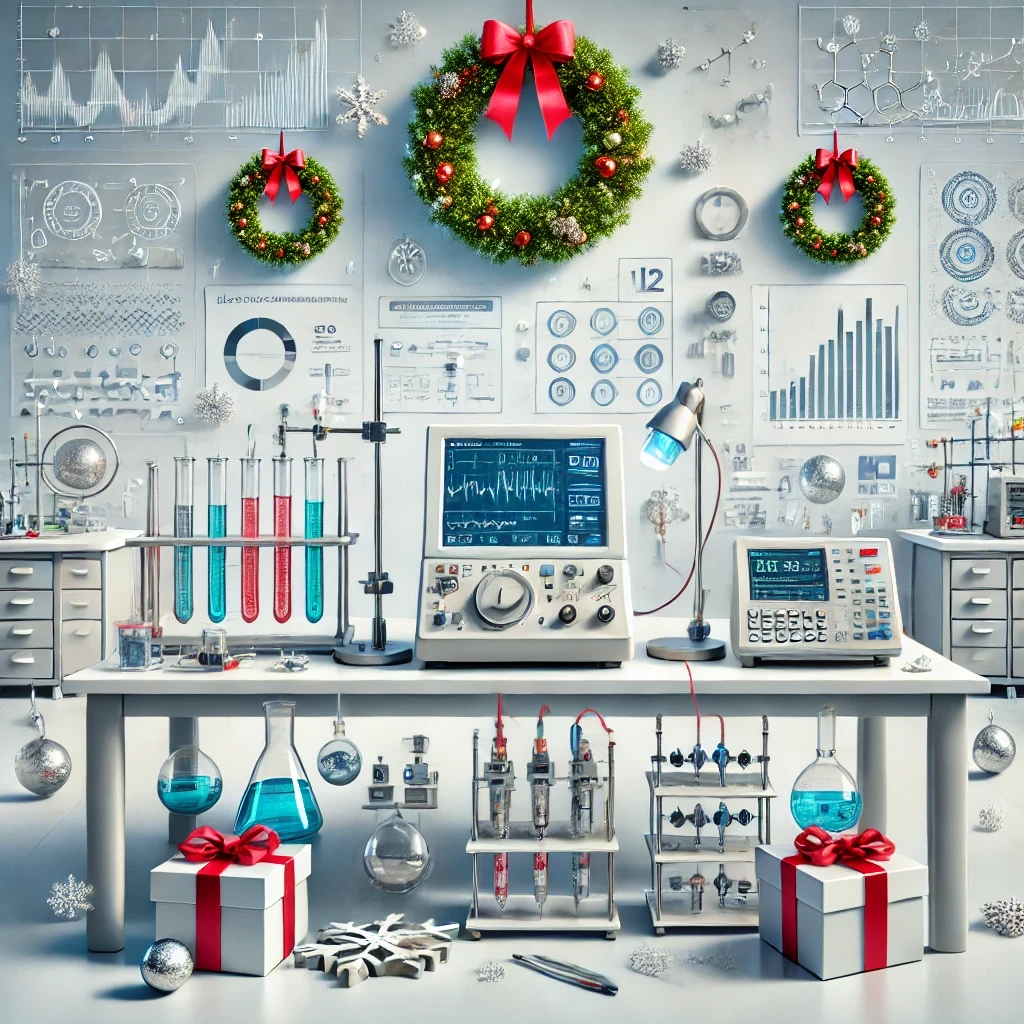
12 Days of Electrochemical Testing
To celebrate the holiday season and the re-release of Ionworks Studio, we featured "12 (business) days of electrochemical testing". Each day we pick a test, give a little bit of information about it, and show you how to run it in Ionworks. 🔋 🎄
Dec 17, 2024
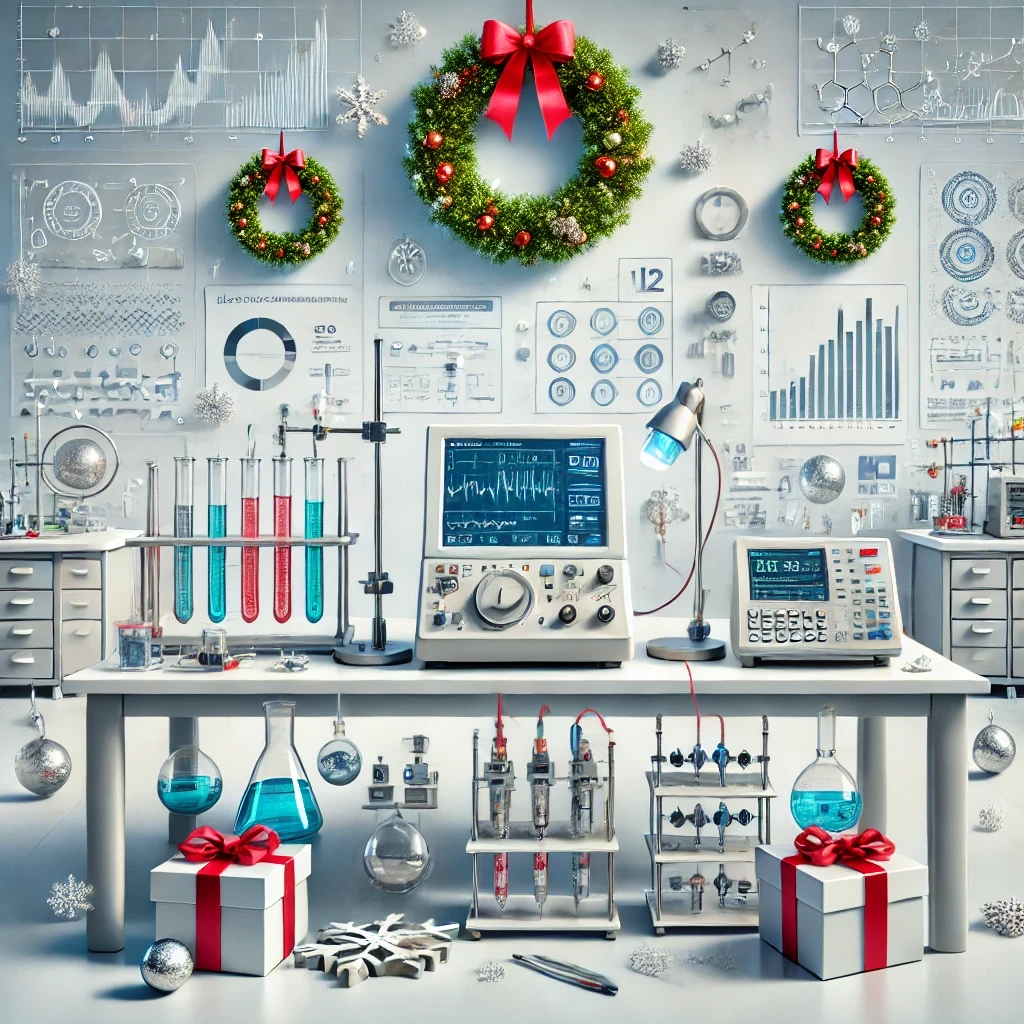
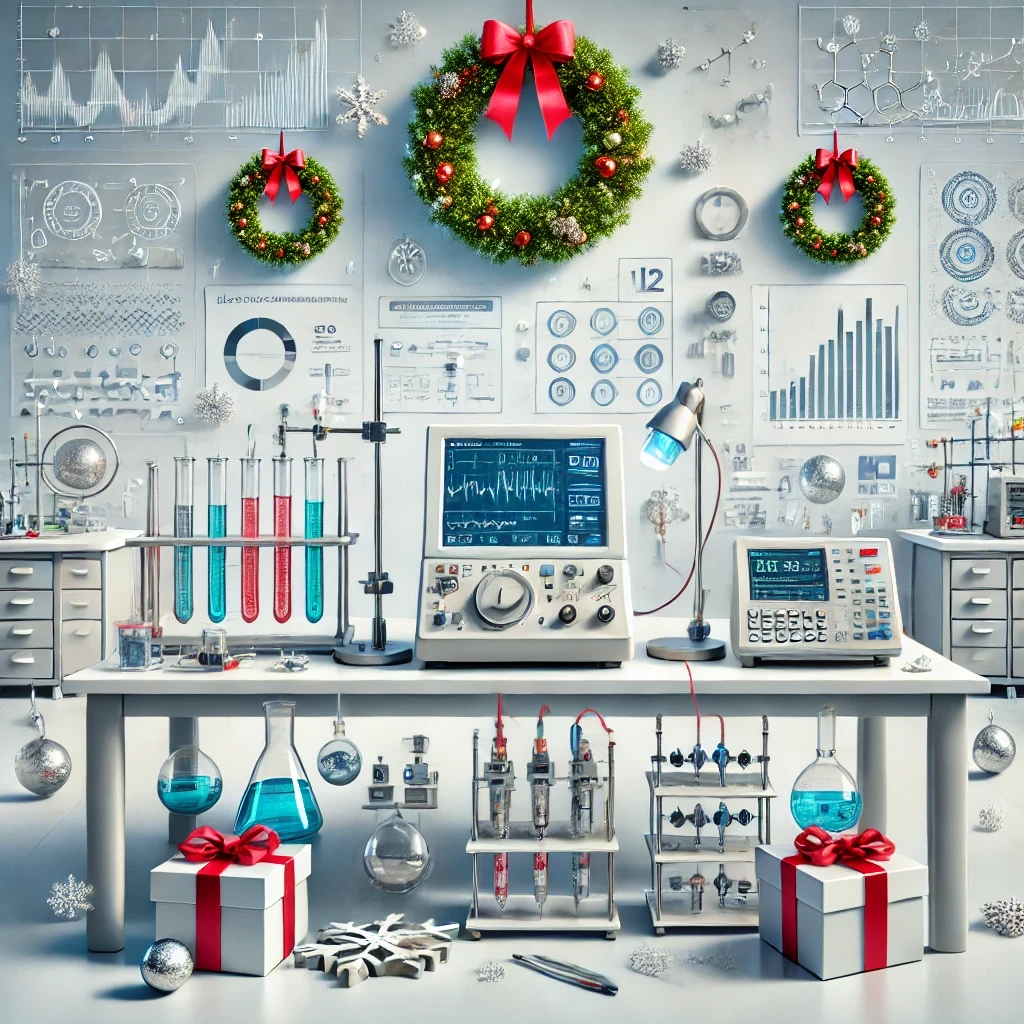
12 Days of Electrochemical Testing
To celebrate the holiday season and the re-release of Ionworks Studio, we featured "12 (business) days of electrochemical testing". Each day we pick a test, give a little bit of information about it, and show you how to run it in Ionworks. 🔋 🎄
Dec 17, 2024
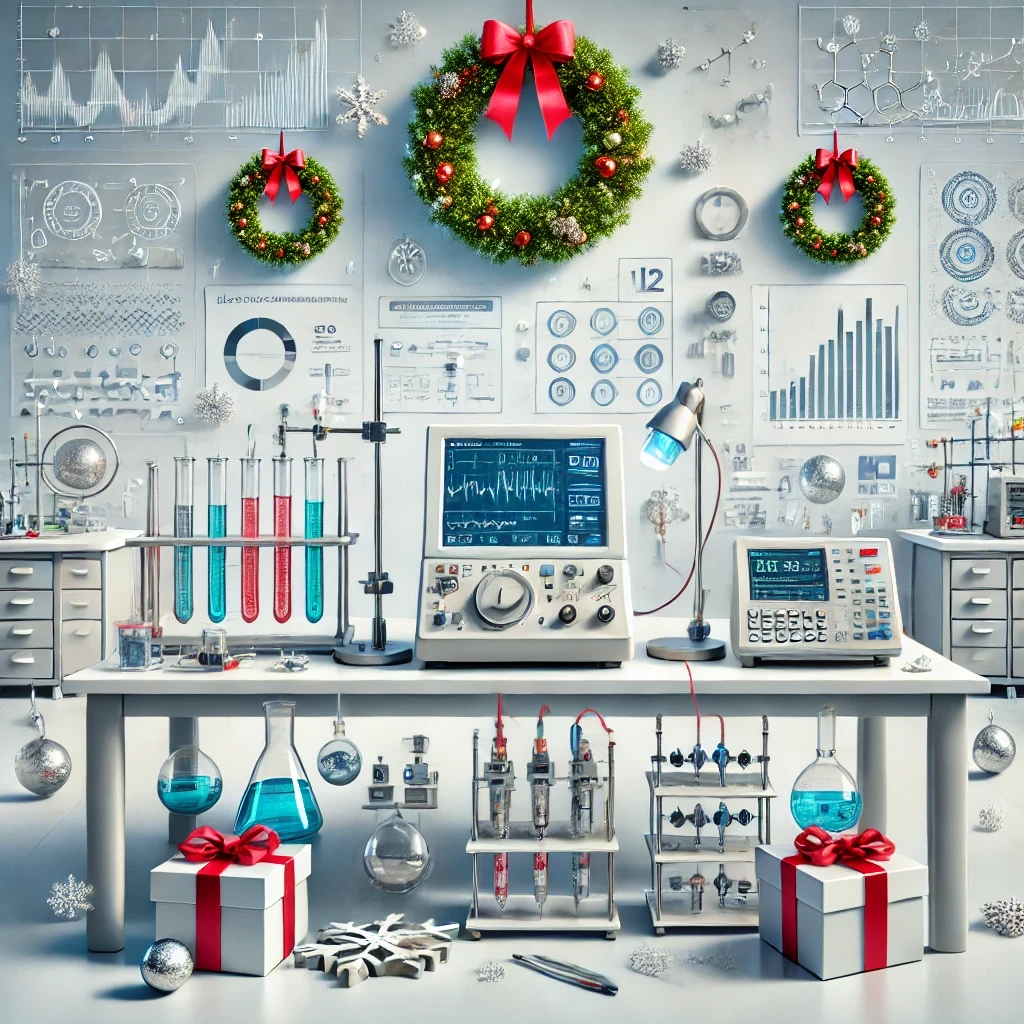
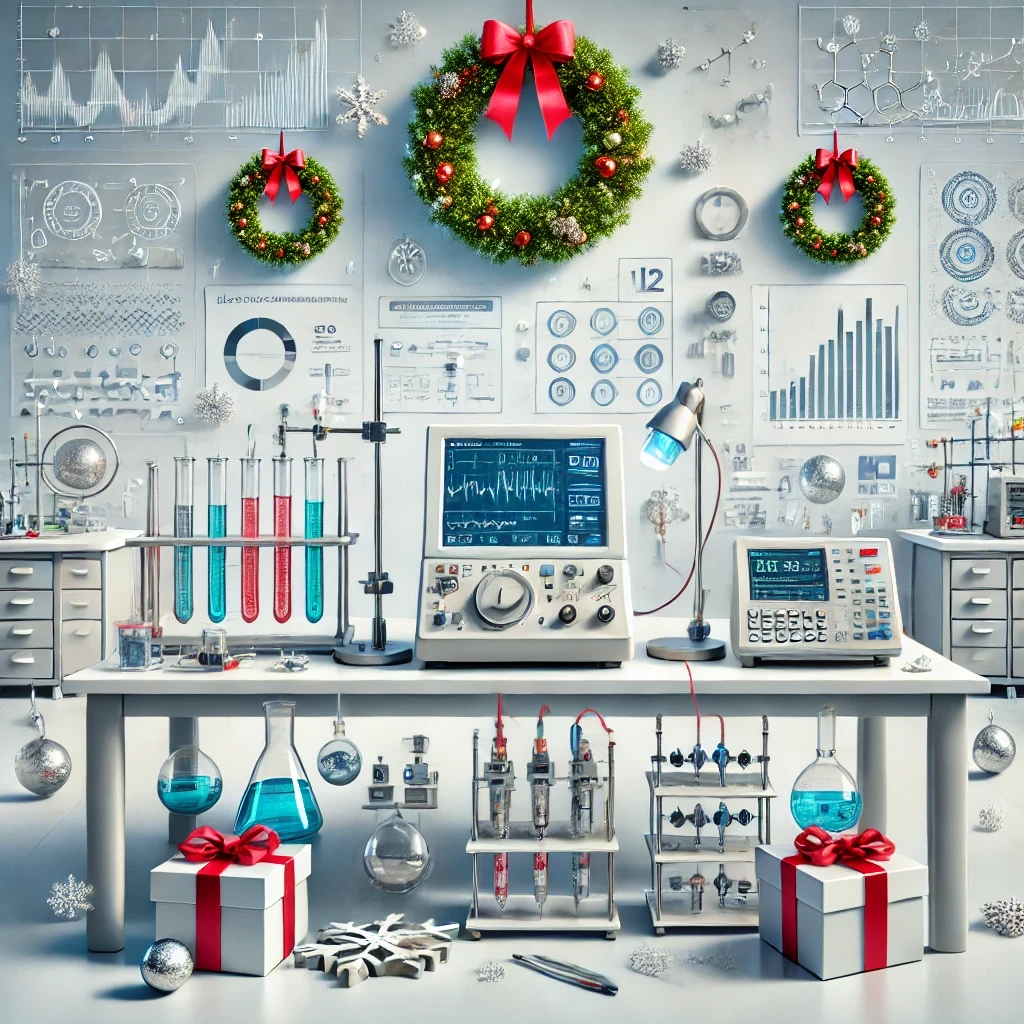
12 Days of Electrochemical Testing
To celebrate the holiday season and the re-release of Ionworks Studio, we featured "12 (business) days of electrochemical testing". Each day we pick a test, give a little bit of information about it, and show you how to run it in Ionworks. 🔋 🎄
Dec 17, 2024
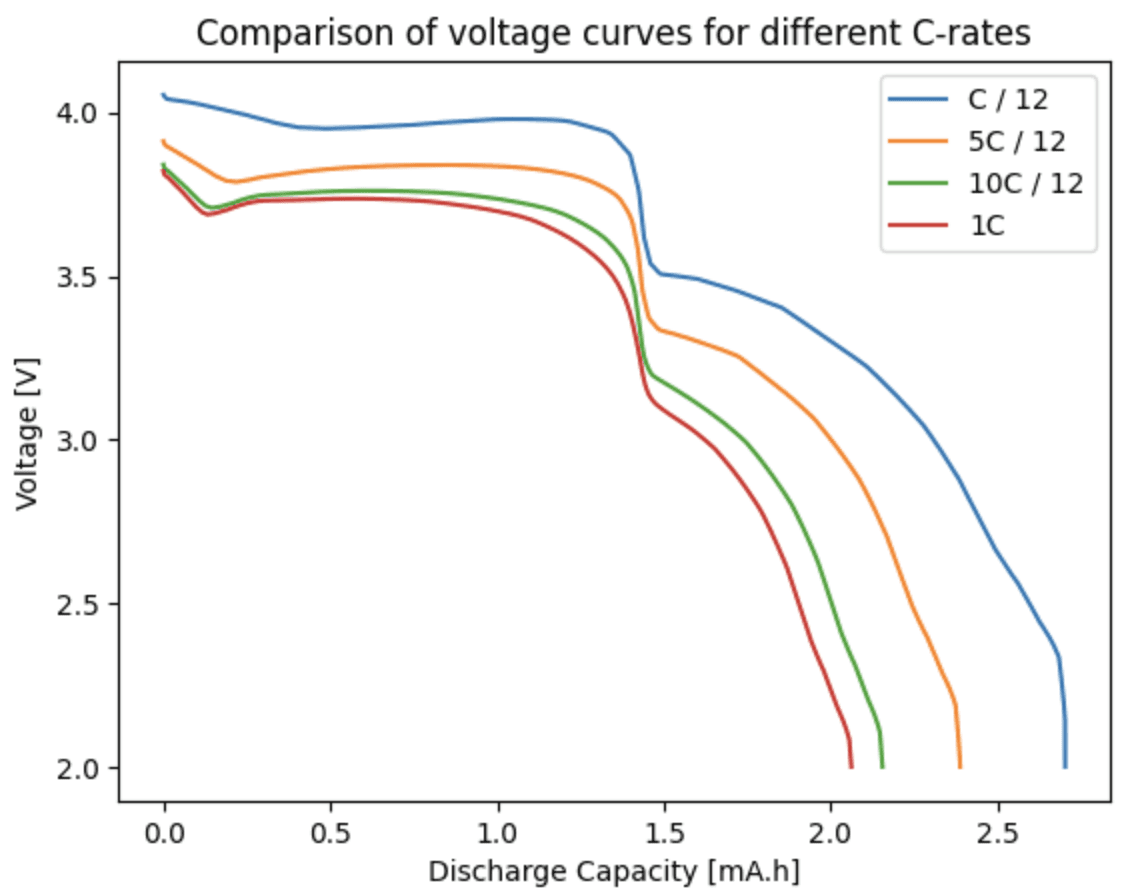
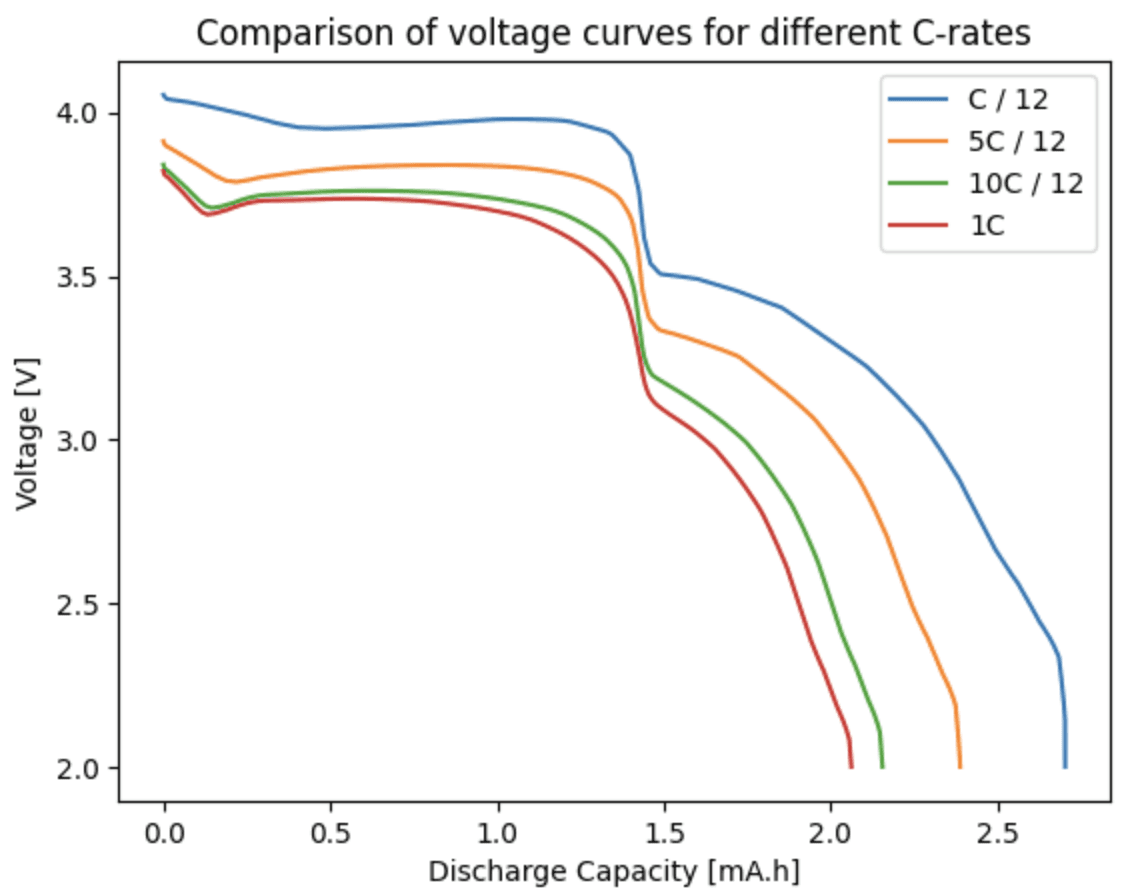
Sodium Ion Battery Model now available in PyBaMM!
This blog post explores the history of SIBs, the intricacies of the new PyBaMM model, and the exciting possibilities it unlocks for the future of energy storage.
Nov 12, 2024
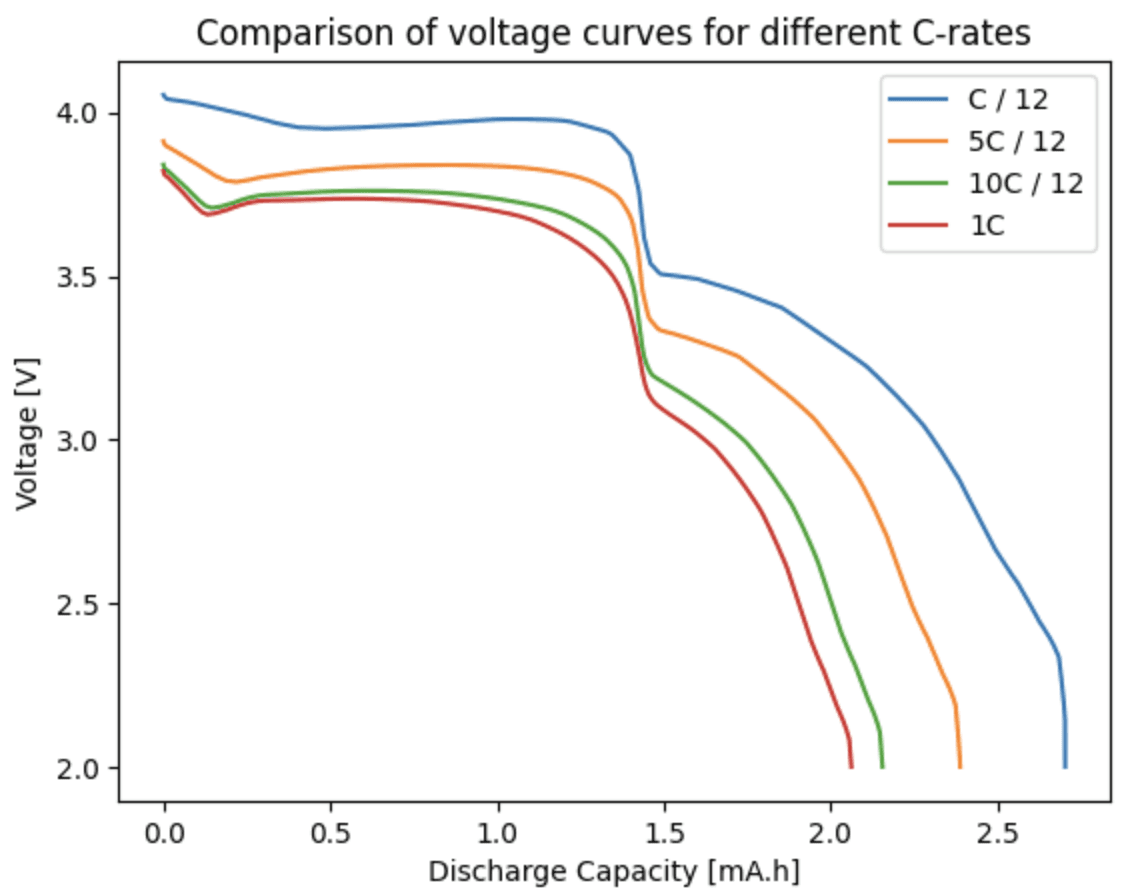
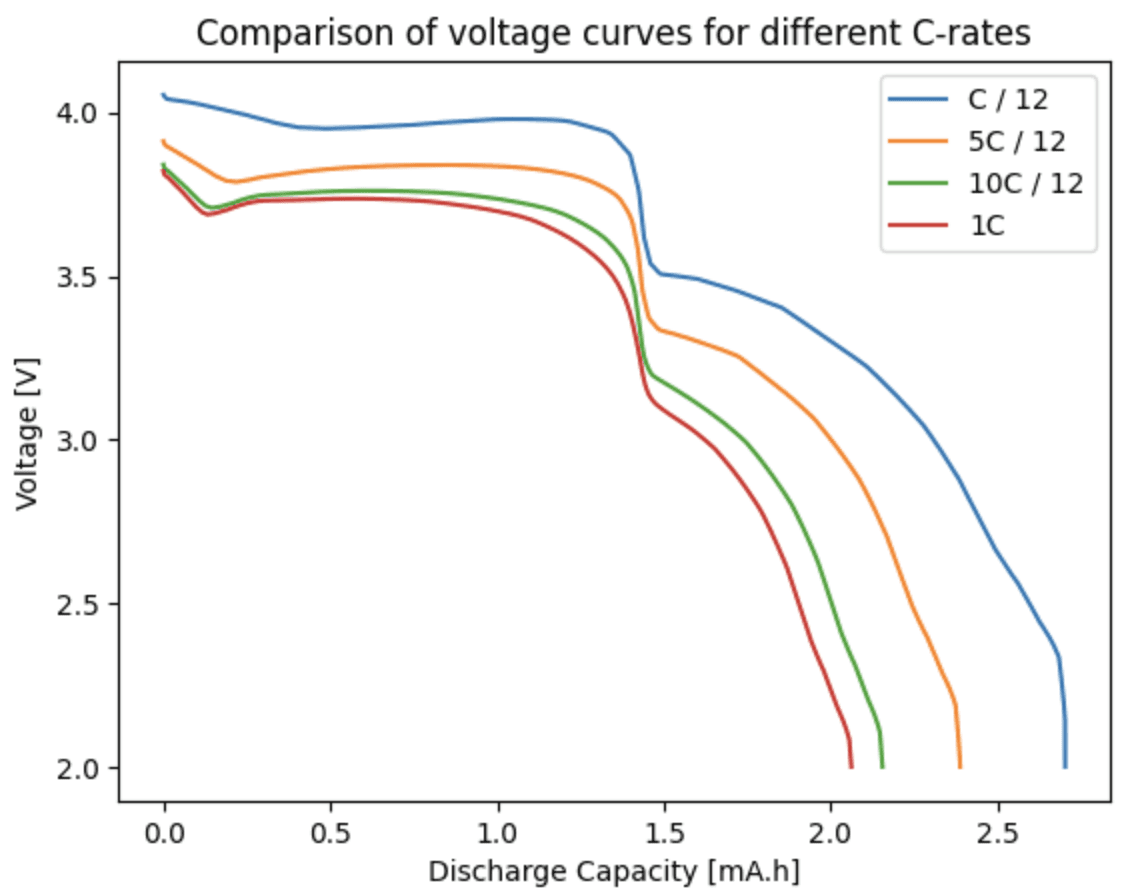
Sodium Ion Battery Model now available in PyBaMM!
This blog post explores the history of SIBs, the intricacies of the new PyBaMM model, and the exciting possibilities it unlocks for the future of energy storage.
Nov 12, 2024
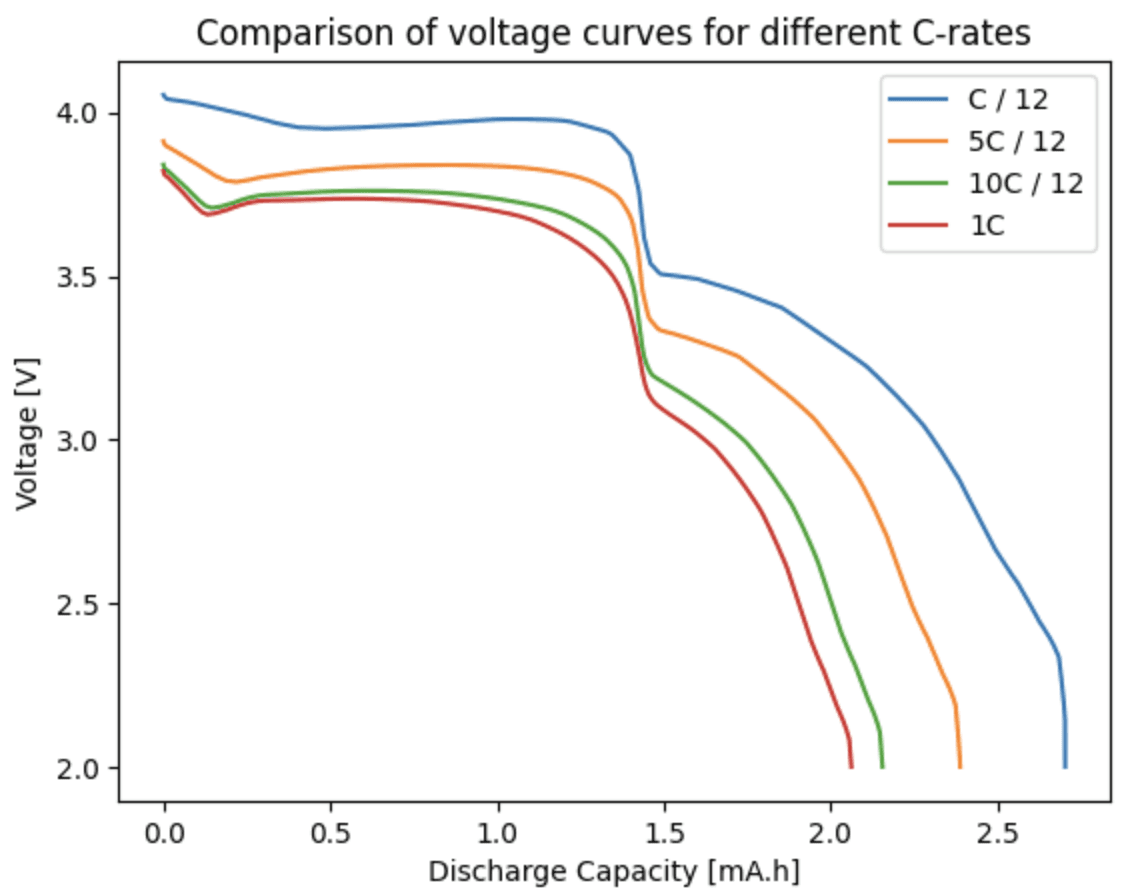
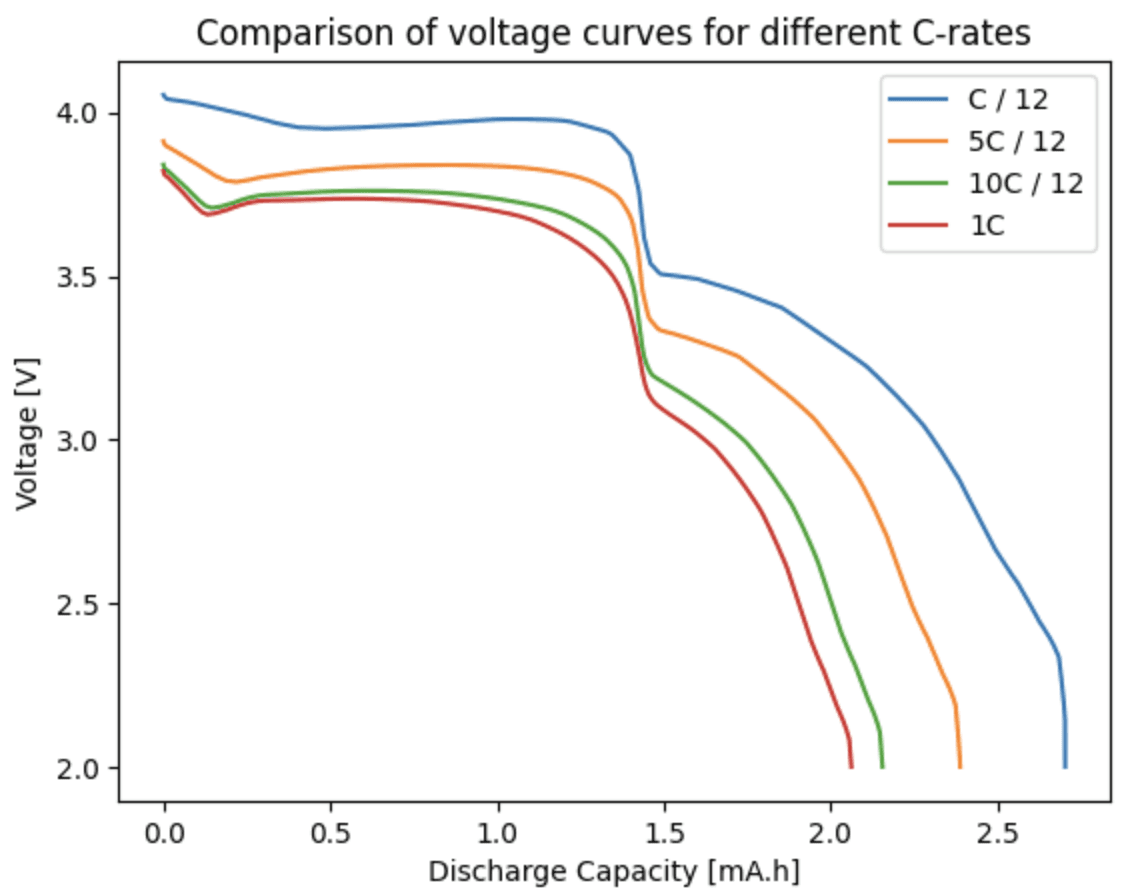
Sodium Ion Battery Model now available in PyBaMM!
This blog post explores the history of SIBs, the intricacies of the new PyBaMM model, and the exciting possibilities it unlocks for the future of energy storage.
Nov 12, 2024
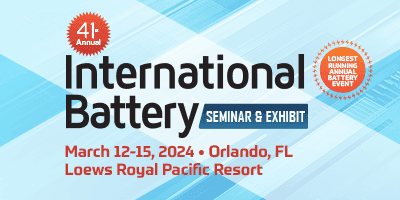
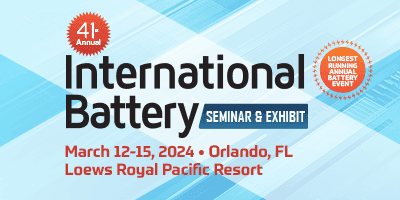
Ionworks Presents at International Battery Seminar
Ionworks CEO Valentin Sulzer presents at the International Battery Seminar in Florida
Mar 12, 2024
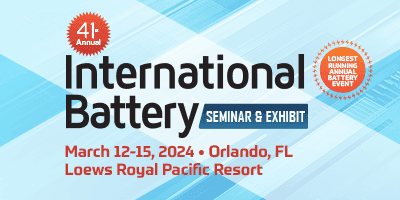
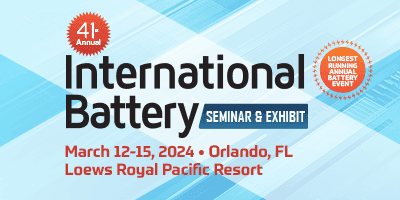
Ionworks Presents at International Battery Seminar
Ionworks CEO Valentin Sulzer presents at the International Battery Seminar in Florida
Mar 12, 2024
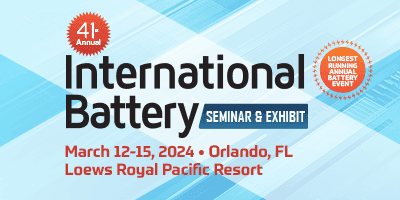
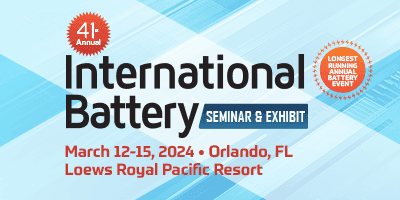
Ionworks Presents at International Battery Seminar
Ionworks CEO Valentin Sulzer presents at the International Battery Seminar in Florida
Mar 12, 2024
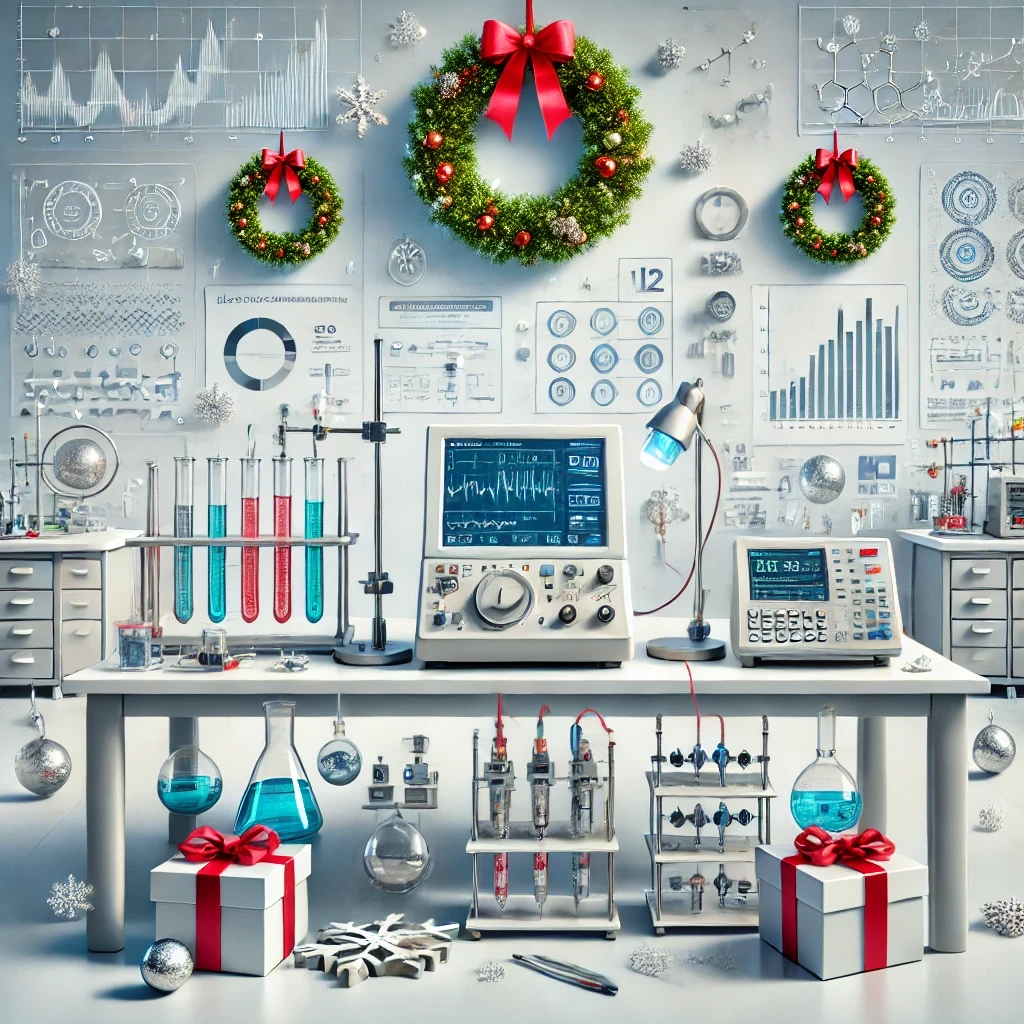
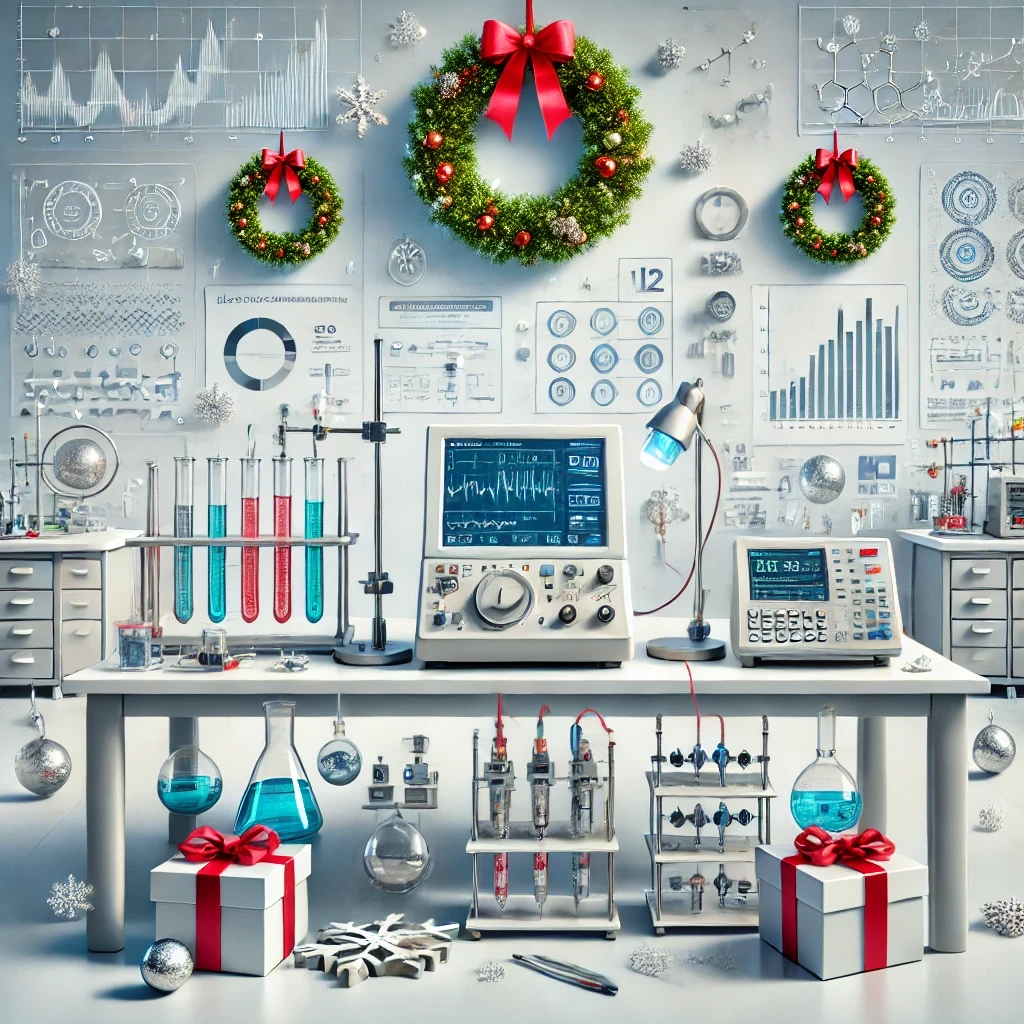
12 Days of Electrochemical Testing
To celebrate the holiday season and the re-release of Ionworks Studio, we featured "12 (business) days of electrochemical testing". Each day we pick a test, give a little bit of information about it, and show you how to run it in Ionworks. 🔋 🎄
Dec 17, 2024
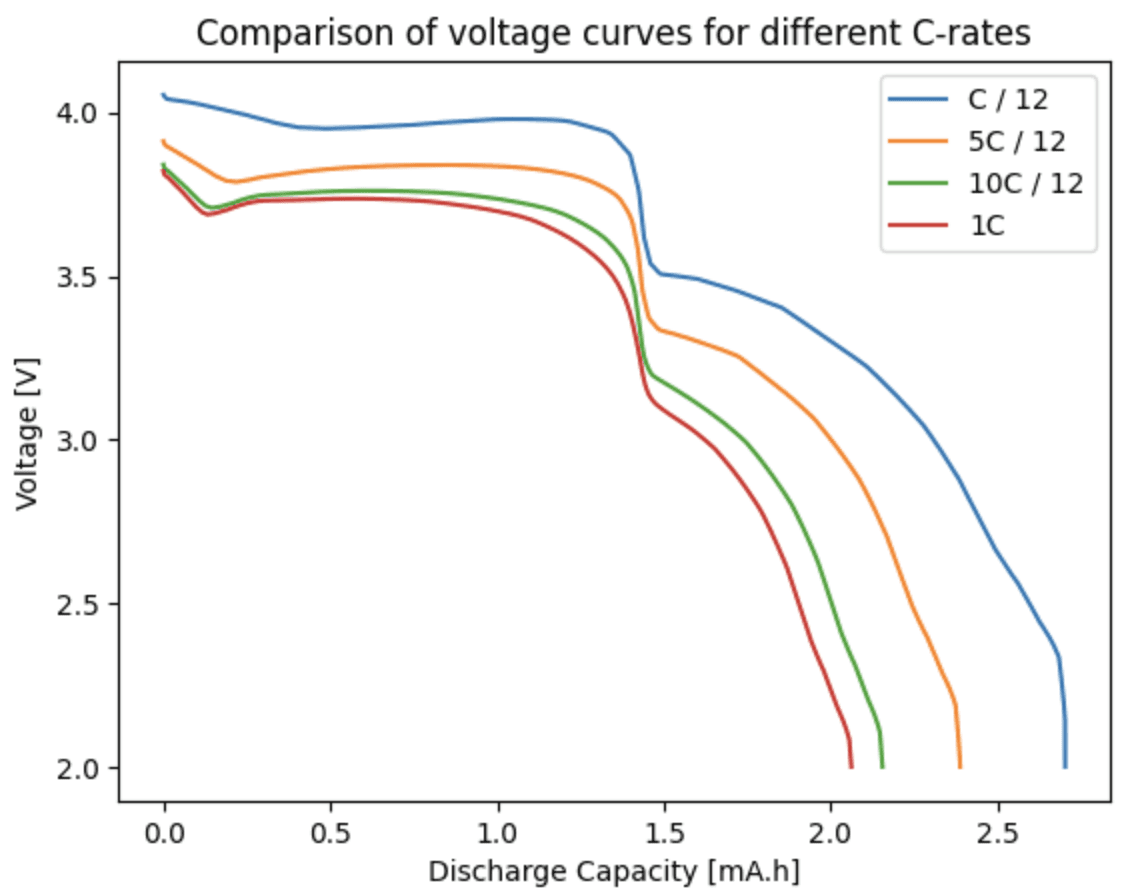
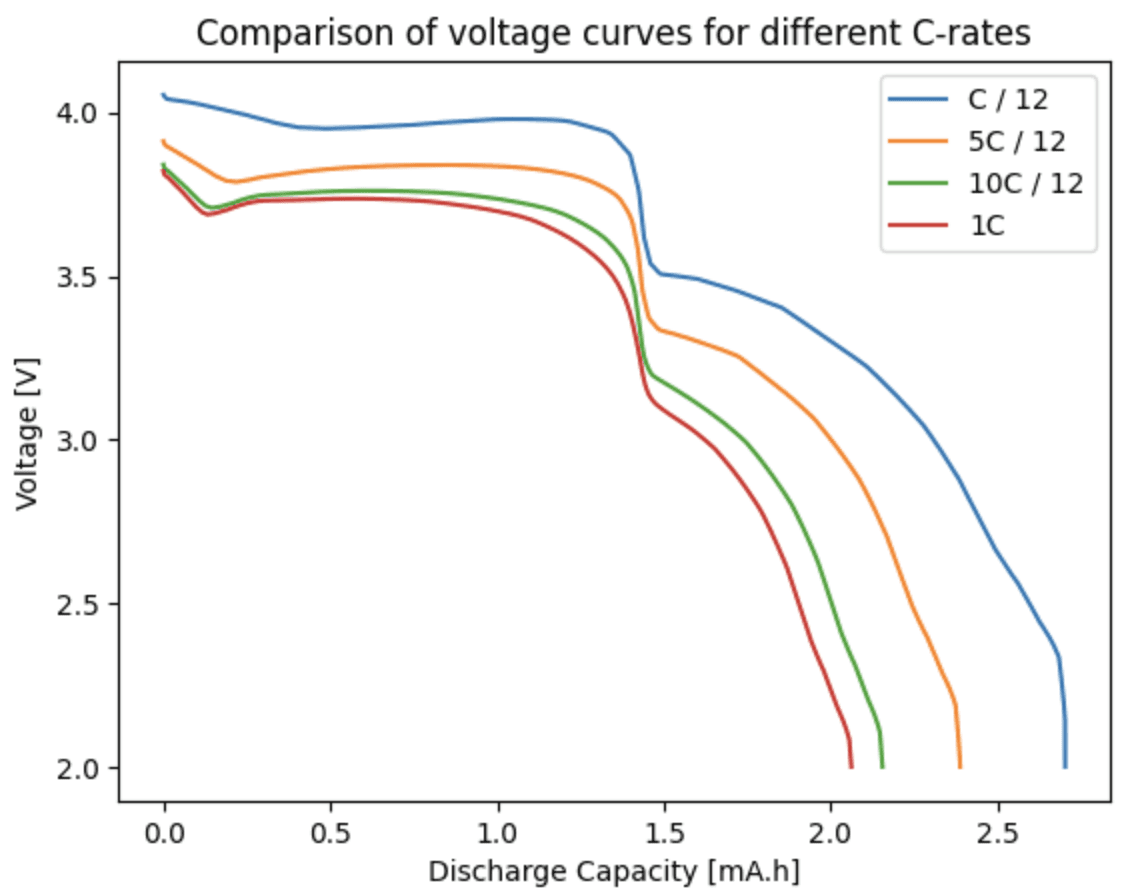
Sodium Ion Battery Model now available in PyBaMM!
This blog post explores the history of SIBs, the intricacies of the new PyBaMM model, and the exciting possibilities it unlocks for the future of energy storage.
Nov 12, 2024
Run your first virtual battery test today
Simulate, iterate, and validate your cell configurations with no lab time required.
Ionworks Technologies Inc. All rights reserved.
Run your first virtual battery test today
Simulate, iterate, and validate your cell configurations with no lab time required.
Run your first virtual battery test today
Simulate, iterate, and validate your cell configurations with no lab time required.
Ionworks Technologies Inc. All rights reserved.
Run your first virtual battery test today
Simulate, iterate, and validate your cell configurations with no lab time required.
Ionworks Technologies Inc. All rights reserved.