Apr 6, 2025
Apr 6, 2025
Battery Internal Resistance: Power Losses, Heat, and Degradation
Battery Internal Resistance: Power Losses, Heat, and Degradation

In our post on State of Charge (SoC), we explored how to determine how much energy a battery has left. But SoC alone doesn’t tell the whole story when it comes to battery performance. Another critical factor is internal resistance, which influences power output, efficiency, and heat generation during operation. Understanding internal resistance is key to optimizing battery performance, prolonging lifespan, and ensuring safe operation.
Internal resistance refers to the opposition a battery presents to the flow of current. Just like any electrical component, a battery isn’t a perfect energy source—it has inherent resistance to the movement of ions in the electrolyte and electrons through the electrodes and current collectors. This resistance causes energy losses, leading to voltage drops under load and heat generation during operation.
Internal resistance isn’t a fixed value; it varies based on factors such as state of charge, temperature, battery chemistry, and aging. As a battery degrades over time, its internal resistance typically increases, leading to greater energy losses and reduced efficiency.
There are several ways to measure internal resistance, with two of the most common methods being:
Direct Current (DC) Method: This involves applying a short pulse of current to the battery and measuring the instantaneous voltage drop. By using Ohm’s Law (R=ΔV/ΔIR), we can estimate the internal resistance. While simple and widely used, this method primarily captures the resistive (Ohmic) component and may not fully account for slower electrochemical effects.
Alternating Current (AC) Method: A more sophisticated method, Electrochemical Impedance Spectroscopy (EIS), applies an AC signal at different frequencies and measures the impedance response of the battery. This method allows for the separation of different resistance contributions, including charge transfer resistance and diffusion-related effects. EIS provides deeper insights into battery aging and performance but requires specialized equipment.
Internal resistance plays a major role in battery performance due to a series of interconnected effects. Higher resistance leads to greater voltage drops under load, reducing the power a battery can deliver. This, in turn, decreases efficiency, as more energy is wasted as heat. The generated heat raises the battery’s temperature, which can accelerate degradation and, in extreme cases, lead to safety issues like thermal runaway.
For these reasons, internal resistance is one of the key metrics monitored by Battery Management Systems (BMS) to optimize performance and maintain safe operation.
As we’ve just explained, one of the most significant consequences of internal resistance is heat generation, making it impossible to assume a constant battery temperature. Predicting temperature dynamics is the main goal of thermal models—but that’s a topic for our next post.
In our post on State of Charge (SoC), we explored how to determine how much energy a battery has left. But SoC alone doesn’t tell the whole story when it comes to battery performance. Another critical factor is internal resistance, which influences power output, efficiency, and heat generation during operation. Understanding internal resistance is key to optimizing battery performance, prolonging lifespan, and ensuring safe operation.
Internal resistance refers to the opposition a battery presents to the flow of current. Just like any electrical component, a battery isn’t a perfect energy source—it has inherent resistance to the movement of ions in the electrolyte and electrons through the electrodes and current collectors. This resistance causes energy losses, leading to voltage drops under load and heat generation during operation.
Internal resistance isn’t a fixed value; it varies based on factors such as state of charge, temperature, battery chemistry, and aging. As a battery degrades over time, its internal resistance typically increases, leading to greater energy losses and reduced efficiency.
There are several ways to measure internal resistance, with two of the most common methods being:
Direct Current (DC) Method: This involves applying a short pulse of current to the battery and measuring the instantaneous voltage drop. By using Ohm’s Law (R=ΔV/ΔIR), we can estimate the internal resistance. While simple and widely used, this method primarily captures the resistive (Ohmic) component and may not fully account for slower electrochemical effects.
Alternating Current (AC) Method: A more sophisticated method, Electrochemical Impedance Spectroscopy (EIS), applies an AC signal at different frequencies and measures the impedance response of the battery. This method allows for the separation of different resistance contributions, including charge transfer resistance and diffusion-related effects. EIS provides deeper insights into battery aging and performance but requires specialized equipment.
Internal resistance plays a major role in battery performance due to a series of interconnected effects. Higher resistance leads to greater voltage drops under load, reducing the power a battery can deliver. This, in turn, decreases efficiency, as more energy is wasted as heat. The generated heat raises the battery’s temperature, which can accelerate degradation and, in extreme cases, lead to safety issues like thermal runaway.
For these reasons, internal resistance is one of the key metrics monitored by Battery Management Systems (BMS) to optimize performance and maintain safe operation.
As we’ve just explained, one of the most significant consequences of internal resistance is heat generation, making it impossible to assume a constant battery temperature. Predicting temperature dynamics is the main goal of thermal models—but that’s a topic for our next post.
In our post on State of Charge (SoC), we explored how to determine how much energy a battery has left. But SoC alone doesn’t tell the whole story when it comes to battery performance. Another critical factor is internal resistance, which influences power output, efficiency, and heat generation during operation. Understanding internal resistance is key to optimizing battery performance, prolonging lifespan, and ensuring safe operation.
Internal resistance refers to the opposition a battery presents to the flow of current. Just like any electrical component, a battery isn’t a perfect energy source—it has inherent resistance to the movement of ions in the electrolyte and electrons through the electrodes and current collectors. This resistance causes energy losses, leading to voltage drops under load and heat generation during operation.
Internal resistance isn’t a fixed value; it varies based on factors such as state of charge, temperature, battery chemistry, and aging. As a battery degrades over time, its internal resistance typically increases, leading to greater energy losses and reduced efficiency.
There are several ways to measure internal resistance, with two of the most common methods being:
Direct Current (DC) Method: This involves applying a short pulse of current to the battery and measuring the instantaneous voltage drop. By using Ohm’s Law (R=ΔV/ΔIR), we can estimate the internal resistance. While simple and widely used, this method primarily captures the resistive (Ohmic) component and may not fully account for slower electrochemical effects.
Alternating Current (AC) Method: A more sophisticated method, Electrochemical Impedance Spectroscopy (EIS), applies an AC signal at different frequencies and measures the impedance response of the battery. This method allows for the separation of different resistance contributions, including charge transfer resistance and diffusion-related effects. EIS provides deeper insights into battery aging and performance but requires specialized equipment.
Internal resistance plays a major role in battery performance due to a series of interconnected effects. Higher resistance leads to greater voltage drops under load, reducing the power a battery can deliver. This, in turn, decreases efficiency, as more energy is wasted as heat. The generated heat raises the battery’s temperature, which can accelerate degradation and, in extreme cases, lead to safety issues like thermal runaway.
For these reasons, internal resistance is one of the key metrics monitored by Battery Management Systems (BMS) to optimize performance and maintain safe operation.
As we’ve just explained, one of the most significant consequences of internal resistance is heat generation, making it impossible to assume a constant battery temperature. Predicting temperature dynamics is the main goal of thermal models—but that’s a topic for our next post.
In our post on State of Charge (SoC), we explored how to determine how much energy a battery has left. But SoC alone doesn’t tell the whole story when it comes to battery performance. Another critical factor is internal resistance, which influences power output, efficiency, and heat generation during operation. Understanding internal resistance is key to optimizing battery performance, prolonging lifespan, and ensuring safe operation.
Internal resistance refers to the opposition a battery presents to the flow of current. Just like any electrical component, a battery isn’t a perfect energy source—it has inherent resistance to the movement of ions in the electrolyte and electrons through the electrodes and current collectors. This resistance causes energy losses, leading to voltage drops under load and heat generation during operation.
Internal resistance isn’t a fixed value; it varies based on factors such as state of charge, temperature, battery chemistry, and aging. As a battery degrades over time, its internal resistance typically increases, leading to greater energy losses and reduced efficiency.
There are several ways to measure internal resistance, with two of the most common methods being:
Direct Current (DC) Method: This involves applying a short pulse of current to the battery and measuring the instantaneous voltage drop. By using Ohm’s Law (R=ΔV/ΔIR), we can estimate the internal resistance. While simple and widely used, this method primarily captures the resistive (Ohmic) component and may not fully account for slower electrochemical effects.
Alternating Current (AC) Method: A more sophisticated method, Electrochemical Impedance Spectroscopy (EIS), applies an AC signal at different frequencies and measures the impedance response of the battery. This method allows for the separation of different resistance contributions, including charge transfer resistance and diffusion-related effects. EIS provides deeper insights into battery aging and performance but requires specialized equipment.
Internal resistance plays a major role in battery performance due to a series of interconnected effects. Higher resistance leads to greater voltage drops under load, reducing the power a battery can deliver. This, in turn, decreases efficiency, as more energy is wasted as heat. The generated heat raises the battery’s temperature, which can accelerate degradation and, in extreme cases, lead to safety issues like thermal runaway.
For these reasons, internal resistance is one of the key metrics monitored by Battery Management Systems (BMS) to optimize performance and maintain safe operation.
As we’ve just explained, one of the most significant consequences of internal resistance is heat generation, making it impossible to assume a constant battery temperature. Predicting temperature dynamics is the main goal of thermal models—but that’s a topic for our next post.
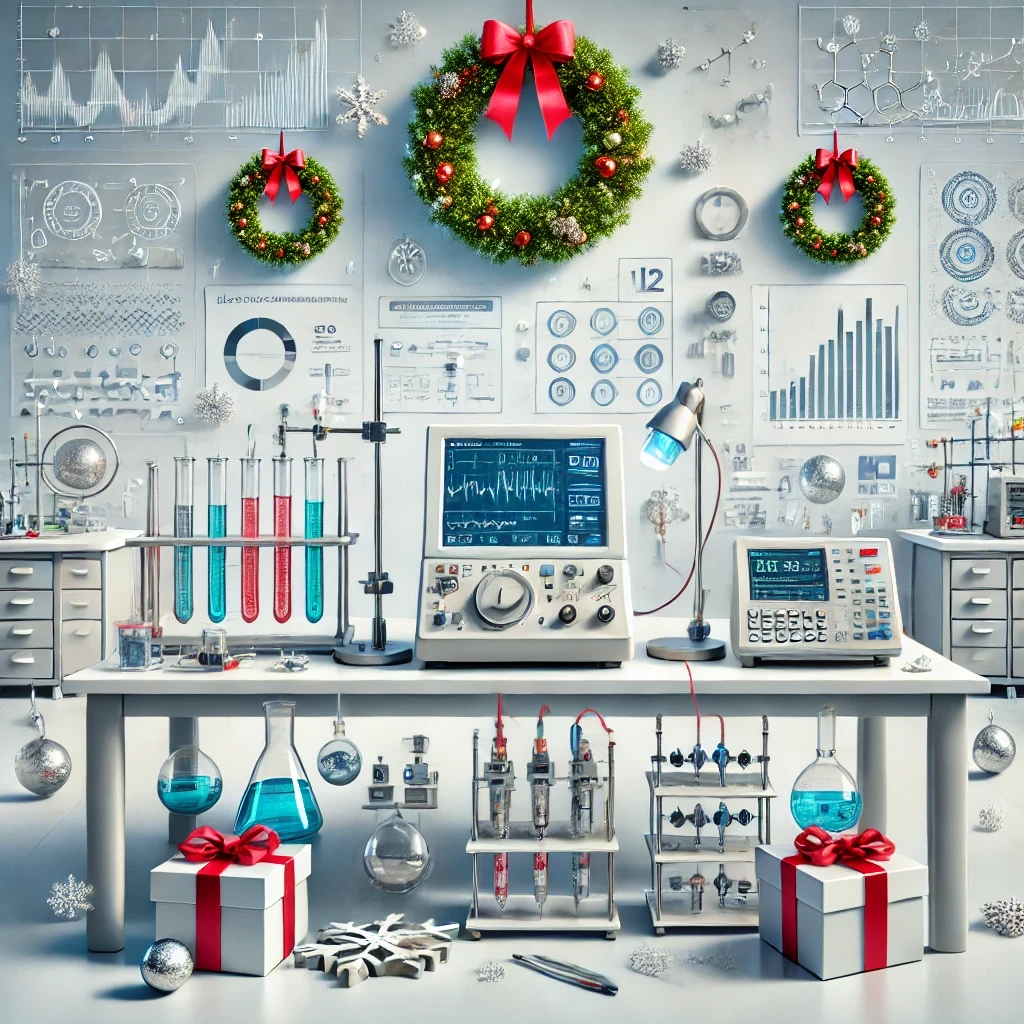
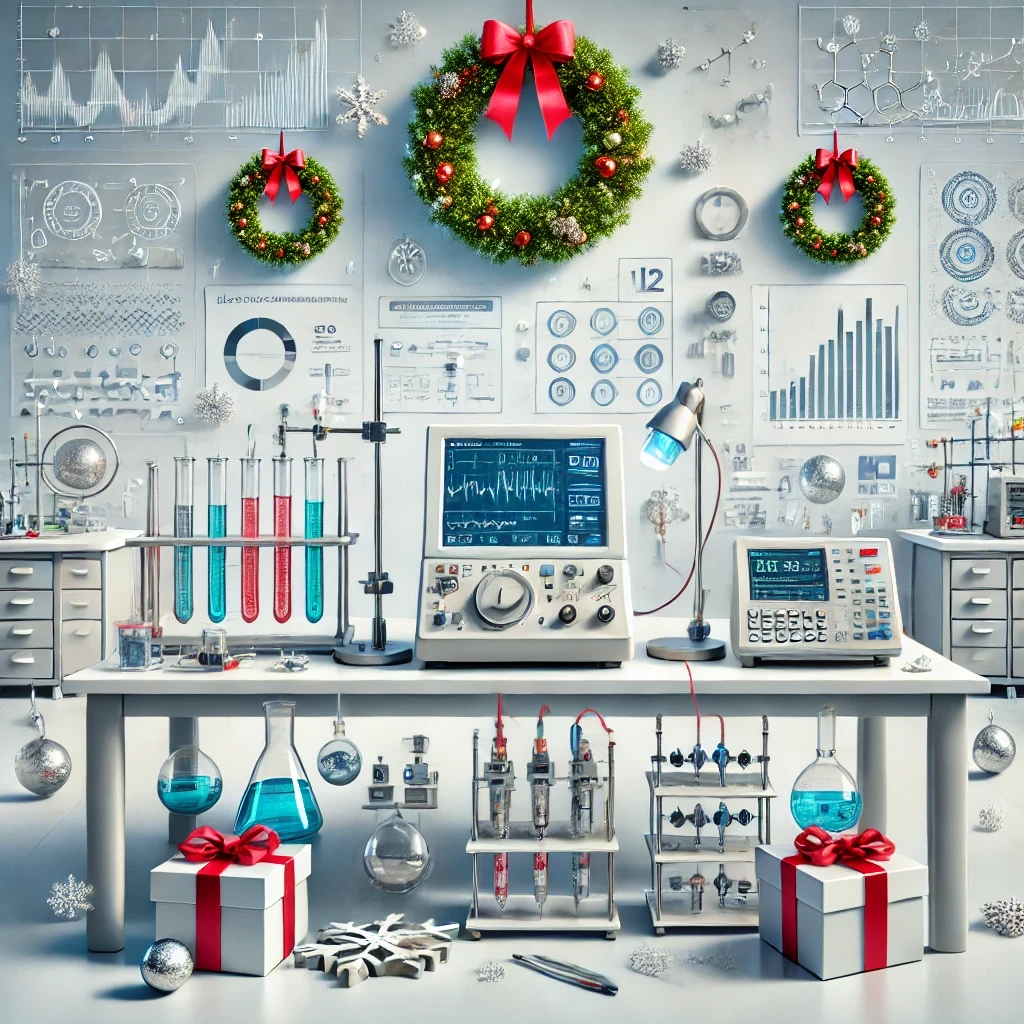
12 Days of Electrochemical Testing
To celebrate the holiday season and the re-release of Ionworks Studio, we featured "12 (business) days of electrochemical testing". Each day we pick a test, give a little bit of information about it, and show you how to run it in Ionworks. 🔋 🎄
Dec 17, 2024
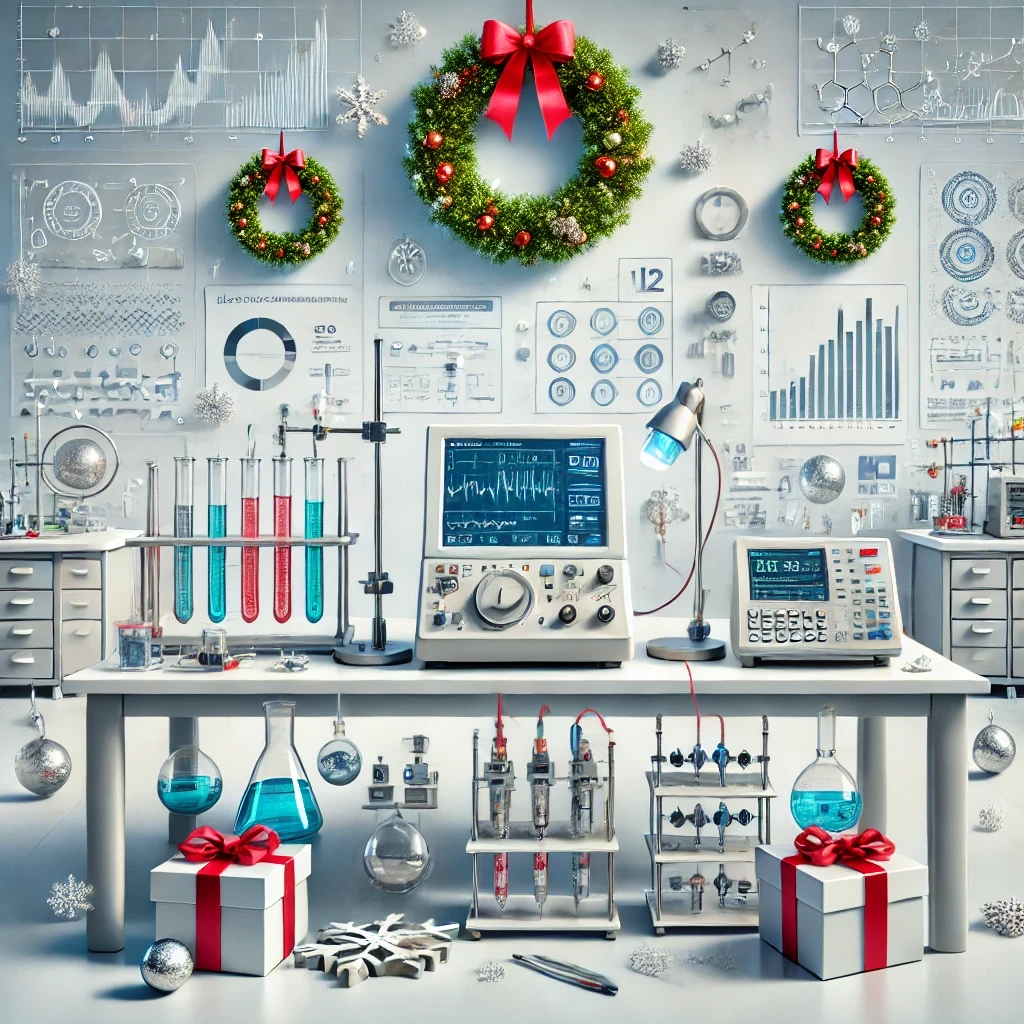
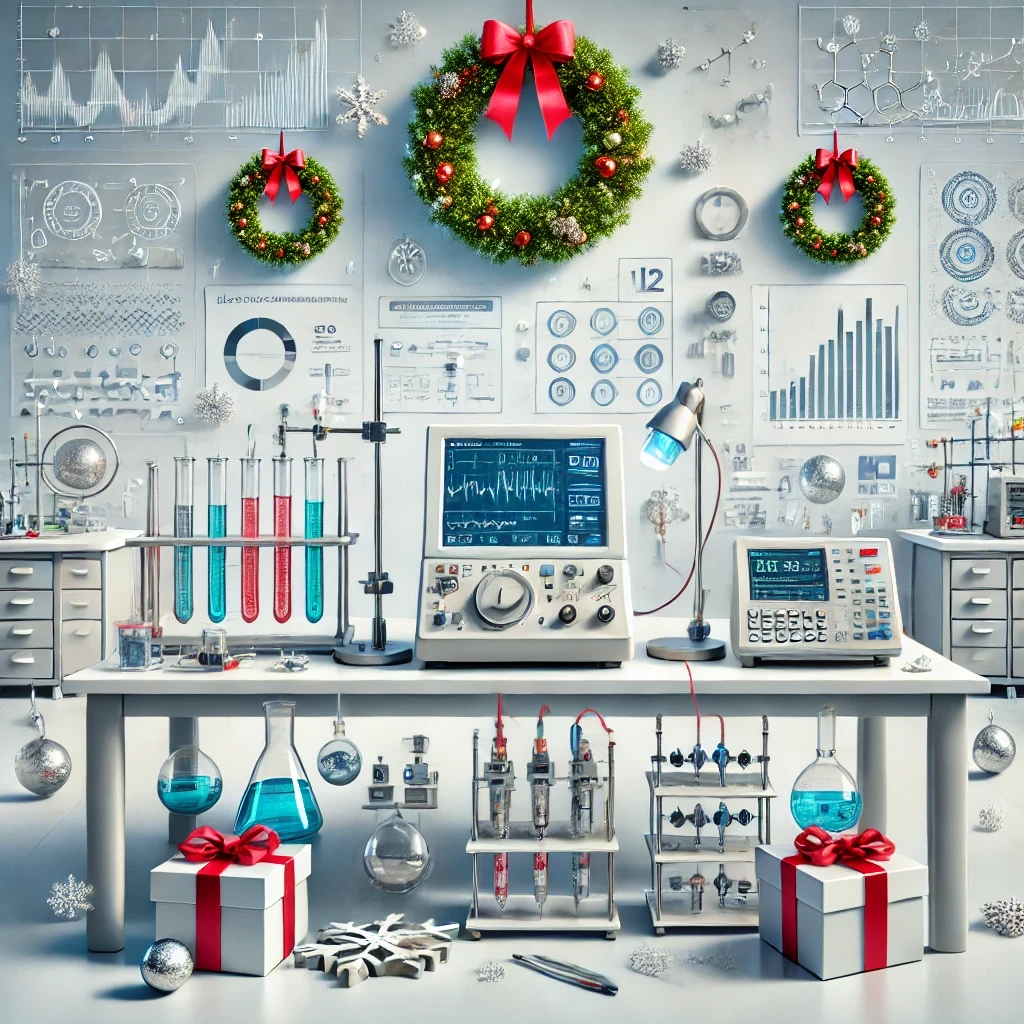
12 Days of Electrochemical Testing
To celebrate the holiday season and the re-release of Ionworks Studio, we featured "12 (business) days of electrochemical testing". Each day we pick a test, give a little bit of information about it, and show you how to run it in Ionworks. 🔋 🎄
Dec 17, 2024
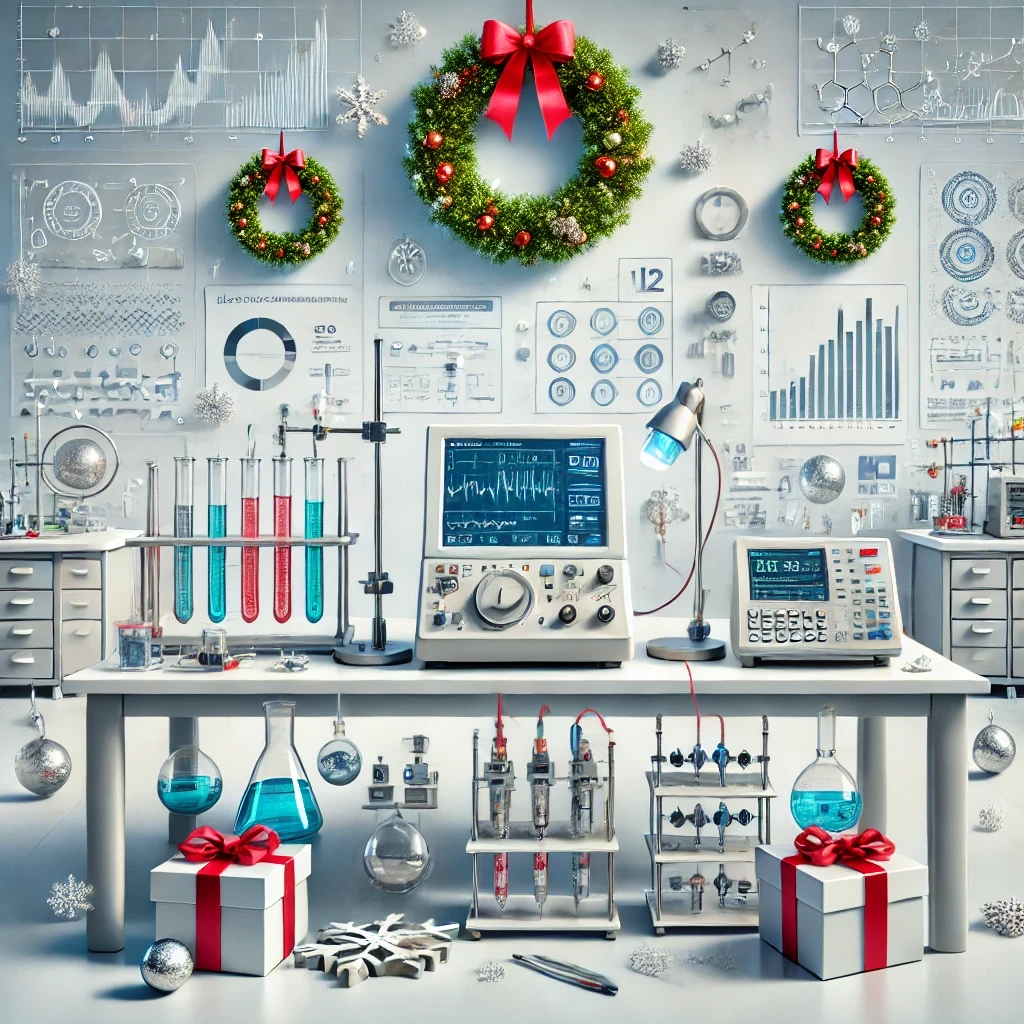
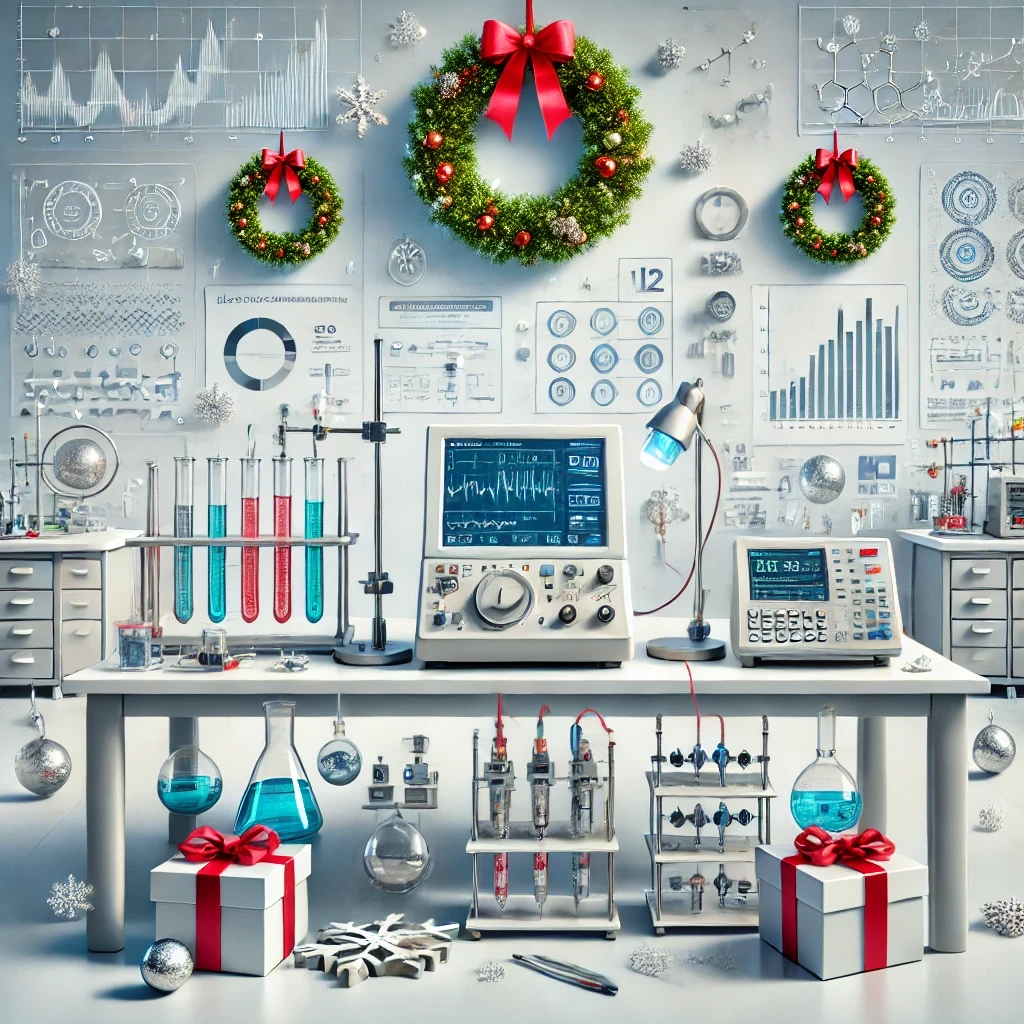
12 Days of Electrochemical Testing
To celebrate the holiday season and the re-release of Ionworks Studio, we featured "12 (business) days of electrochemical testing". Each day we pick a test, give a little bit of information about it, and show you how to run it in Ionworks. 🔋 🎄
Dec 17, 2024
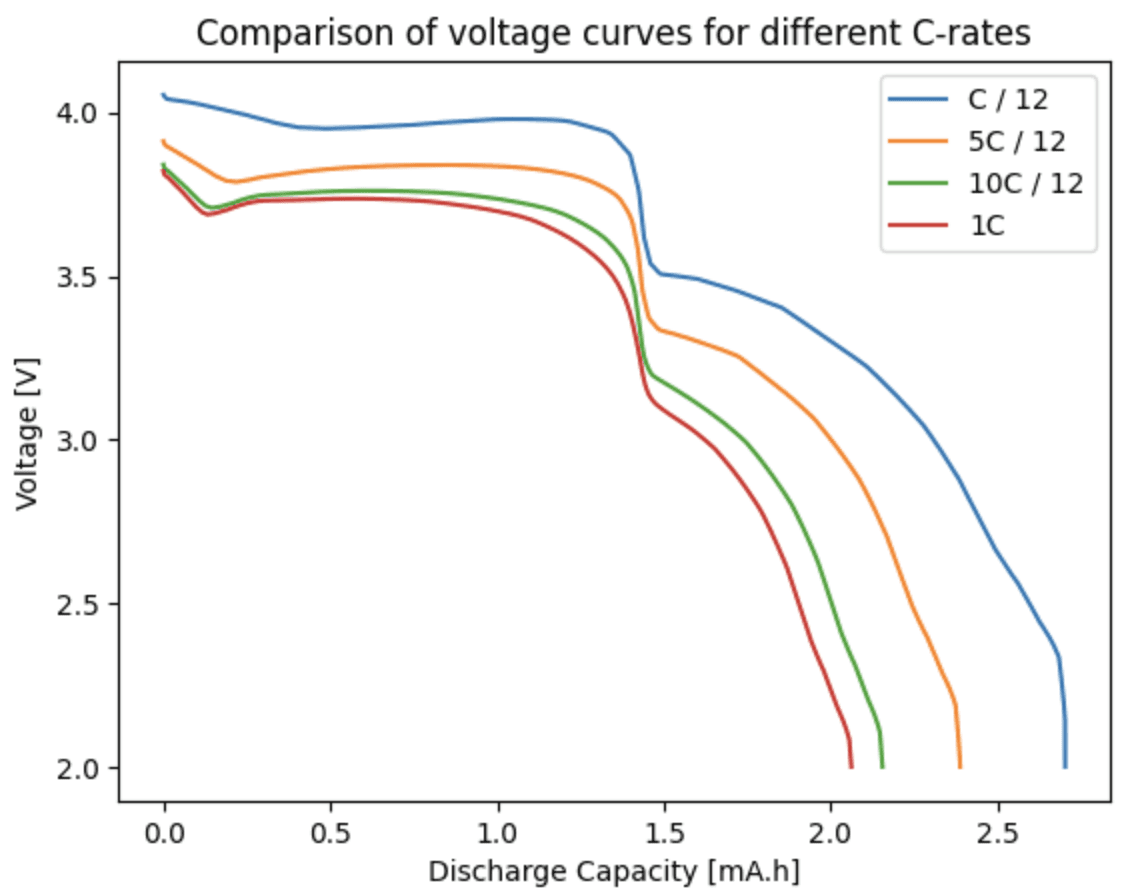
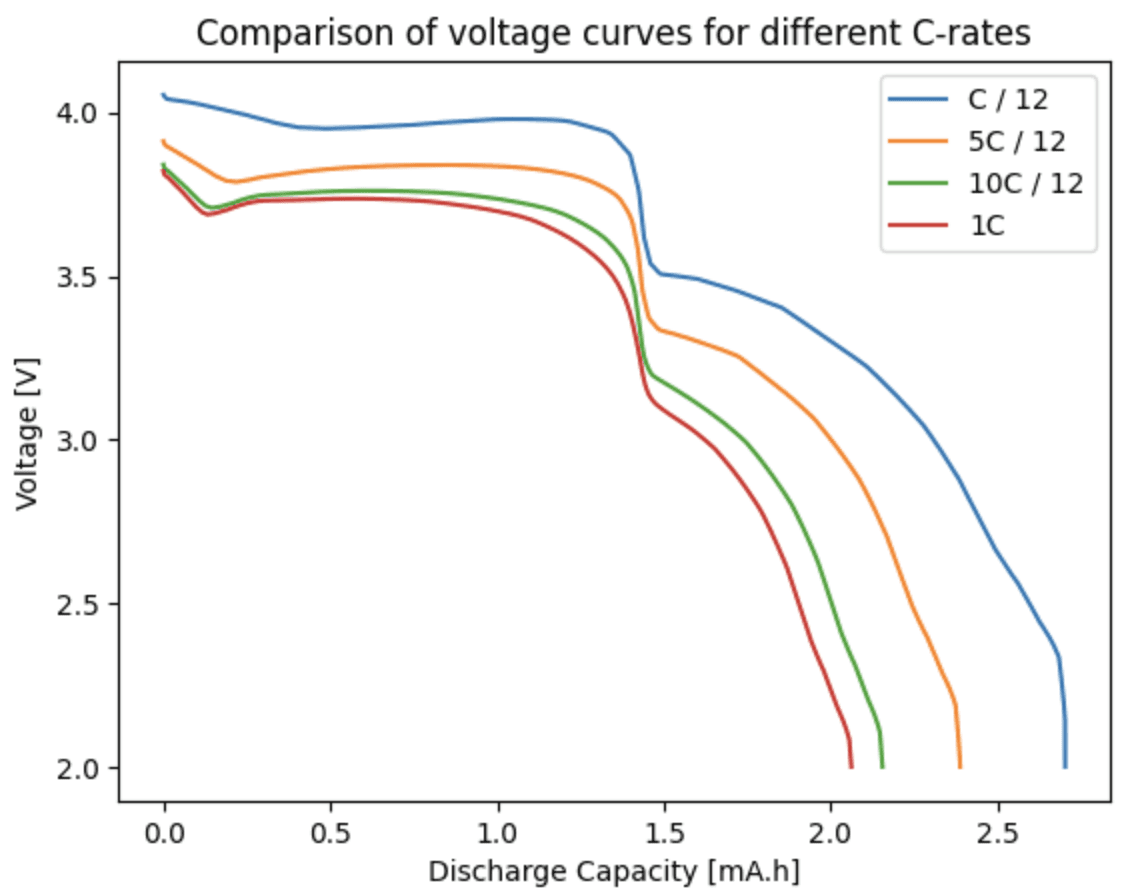
Sodium Ion Battery Model now available in PyBaMM!
This blog post explores the history of SIBs, the intricacies of the new PyBaMM model, and the exciting possibilities it unlocks for the future of energy storage.
Nov 12, 2024
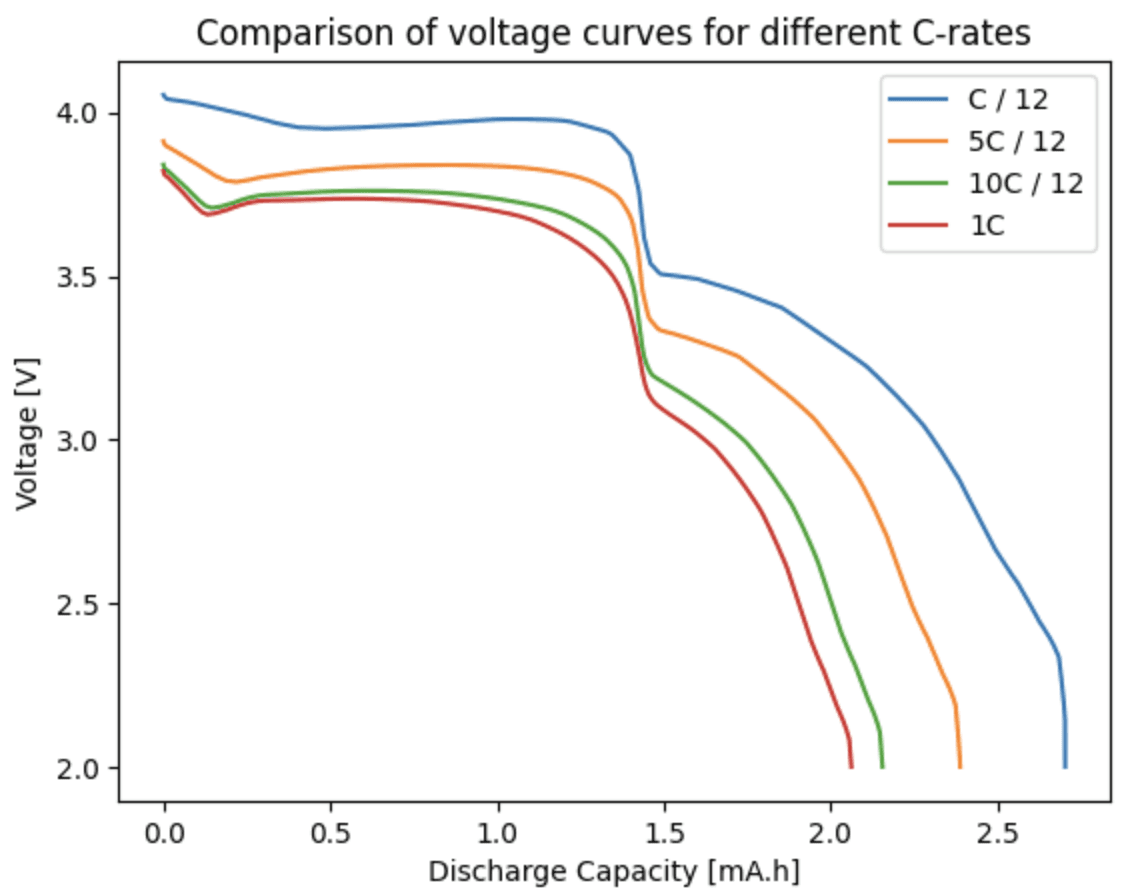
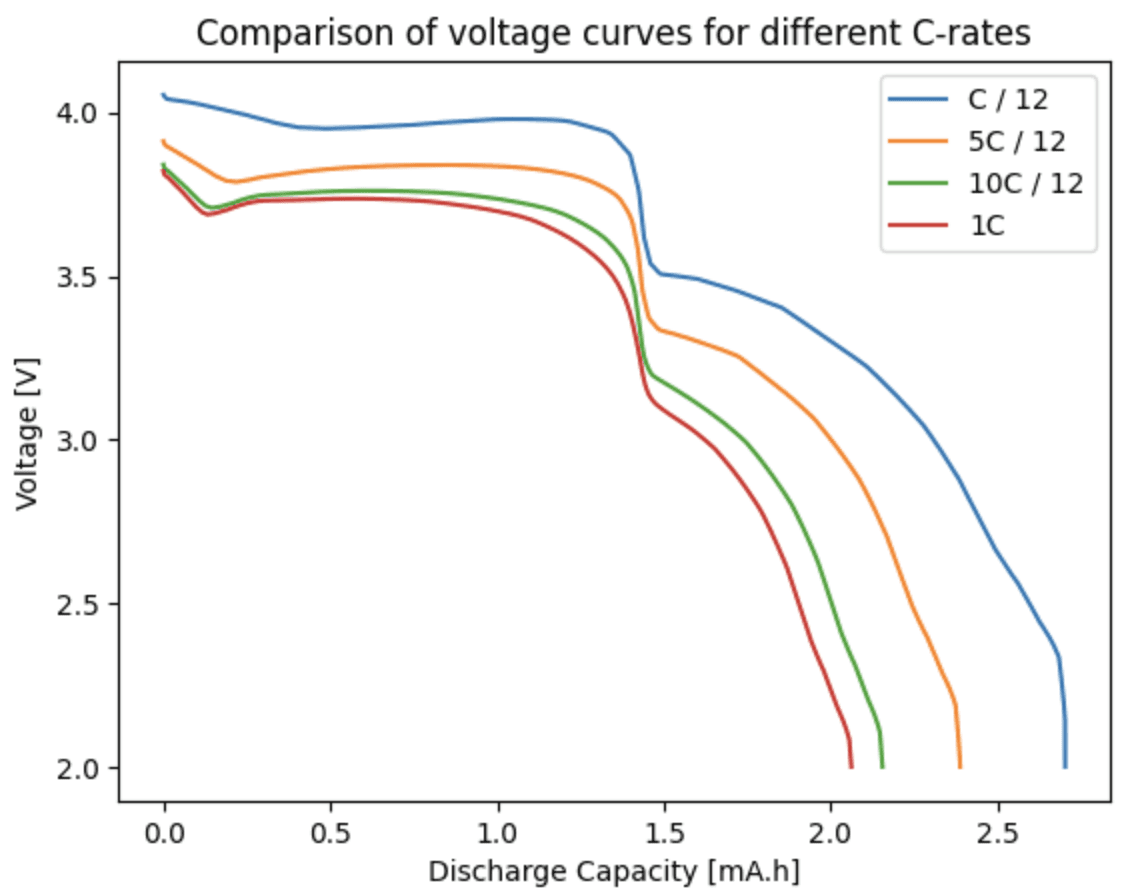
Sodium Ion Battery Model now available in PyBaMM!
This blog post explores the history of SIBs, the intricacies of the new PyBaMM model, and the exciting possibilities it unlocks for the future of energy storage.
Nov 12, 2024
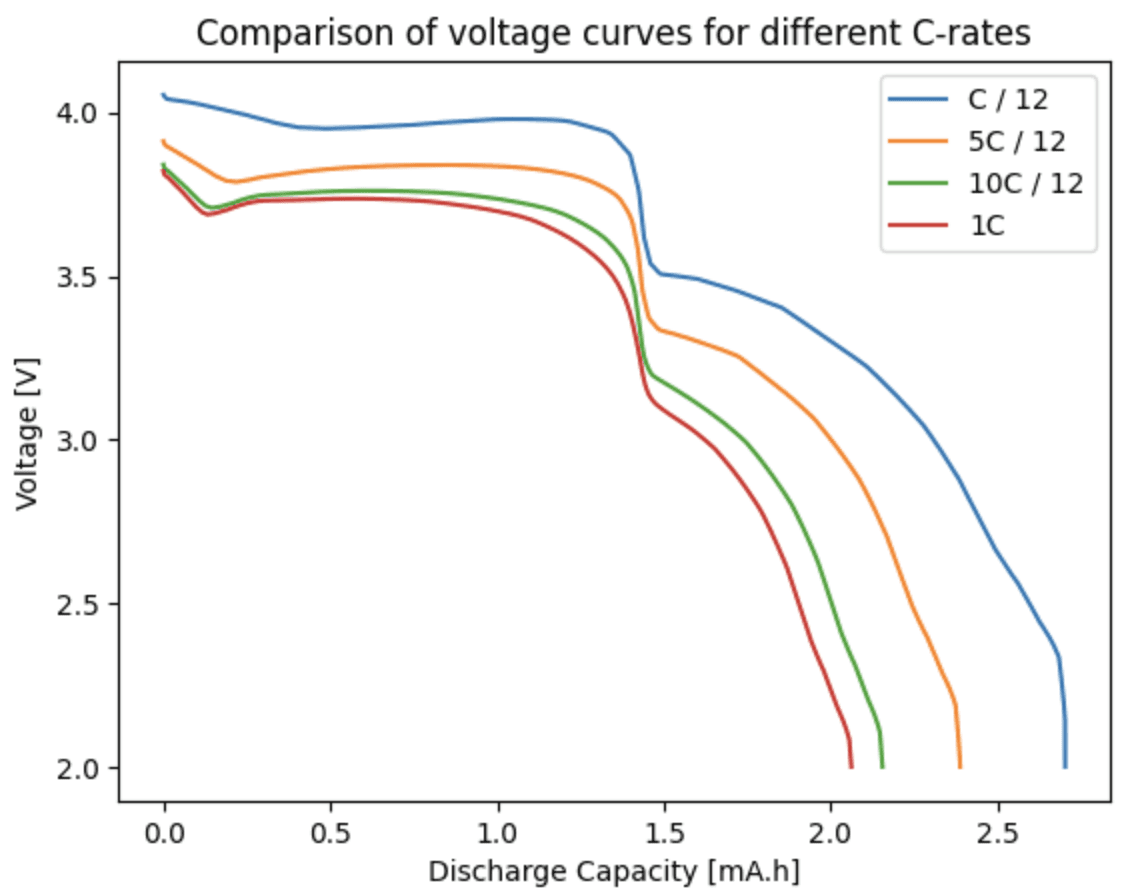
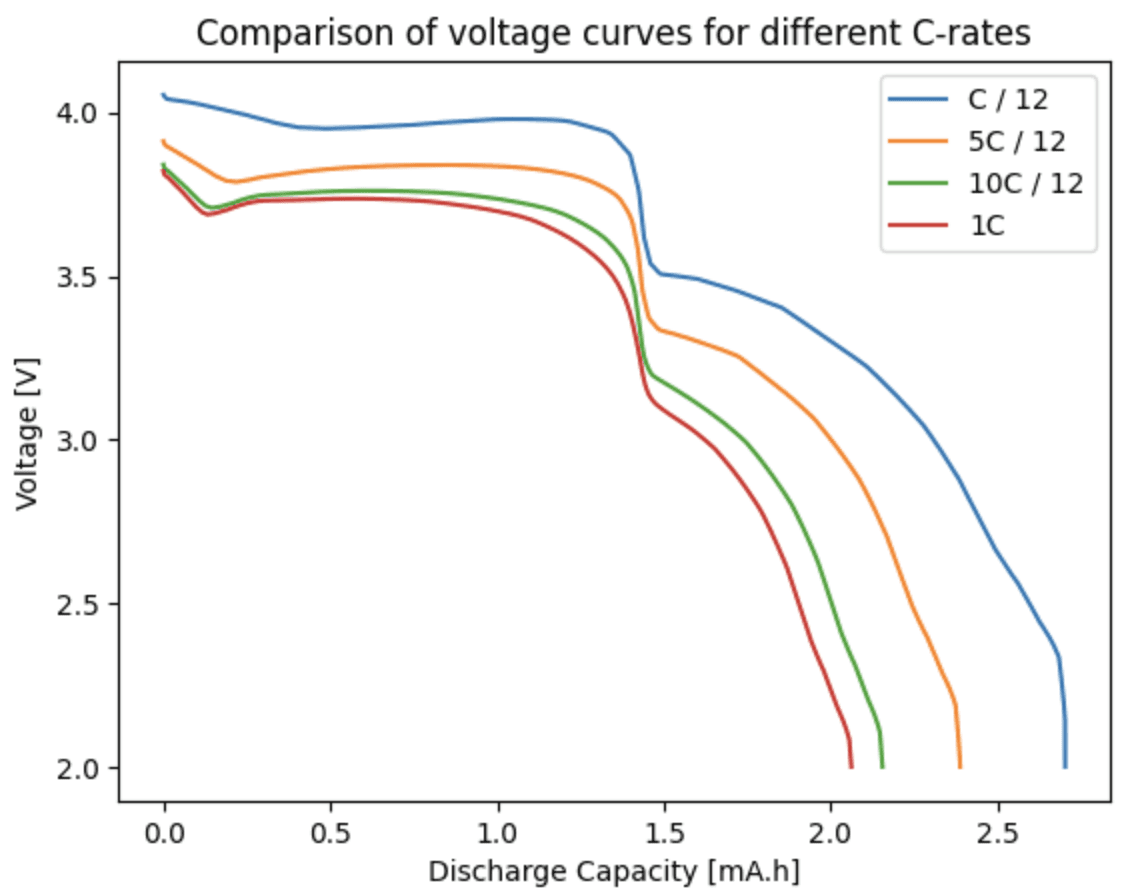
Sodium Ion Battery Model now available in PyBaMM!
This blog post explores the history of SIBs, the intricacies of the new PyBaMM model, and the exciting possibilities it unlocks for the future of energy storage.
Nov 12, 2024
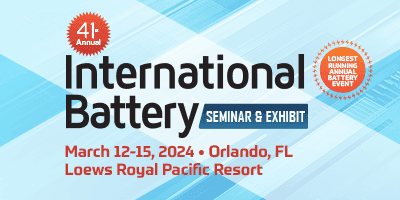
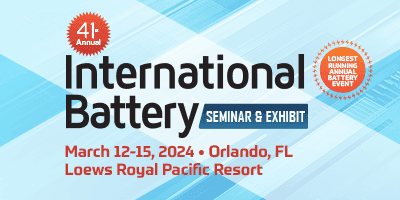
Ionworks Presents at International Battery Seminar
Ionworks CEO Valentin Sulzer presents at the International Battery Seminar in Florida
Mar 12, 2024
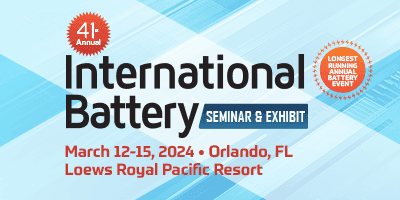
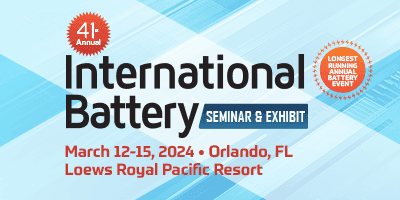
Ionworks Presents at International Battery Seminar
Ionworks CEO Valentin Sulzer presents at the International Battery Seminar in Florida
Mar 12, 2024
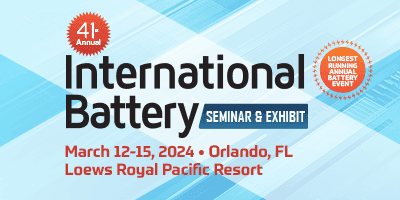
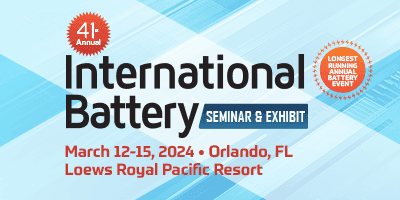
Ionworks Presents at International Battery Seminar
Ionworks CEO Valentin Sulzer presents at the International Battery Seminar in Florida
Mar 12, 2024
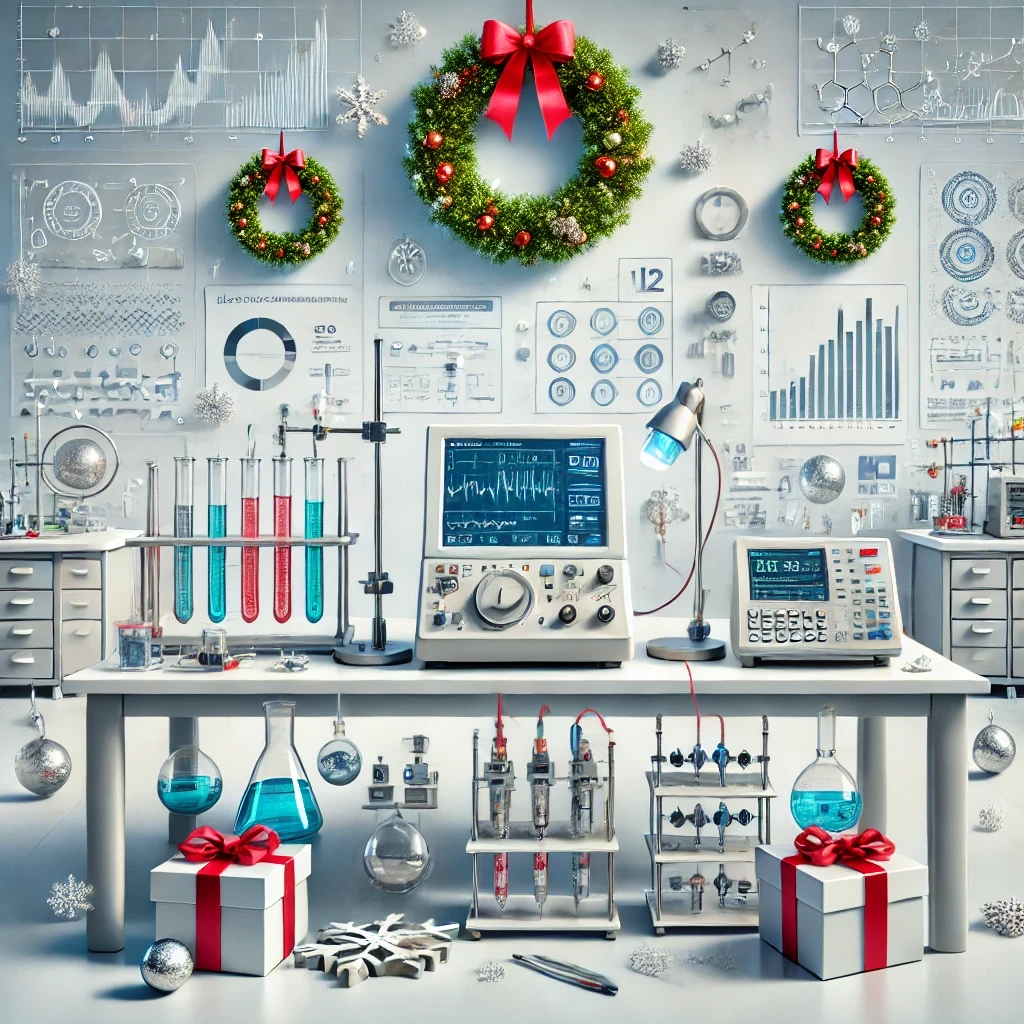
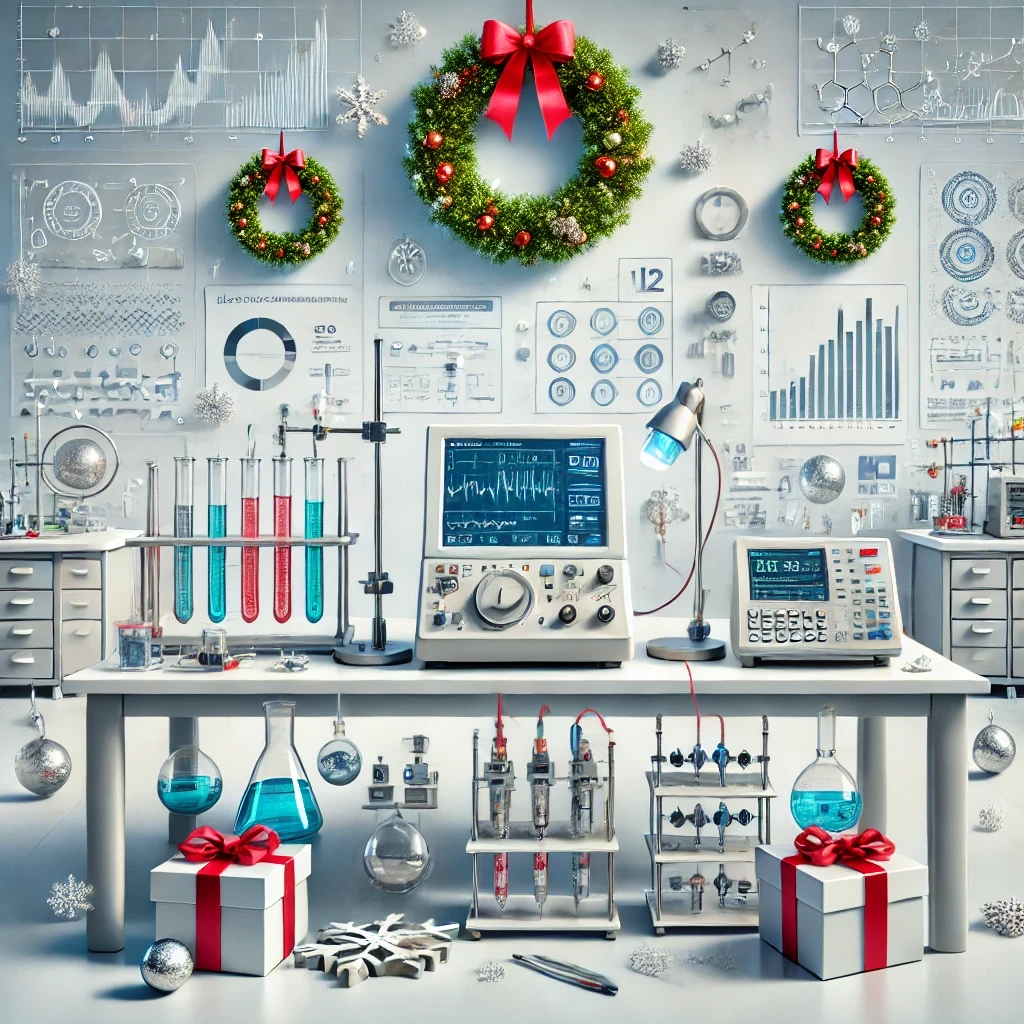
12 Days of Electrochemical Testing
To celebrate the holiday season and the re-release of Ionworks Studio, we featured "12 (business) days of electrochemical testing". Each day we pick a test, give a little bit of information about it, and show you how to run it in Ionworks. 🔋 🎄
Dec 17, 2024
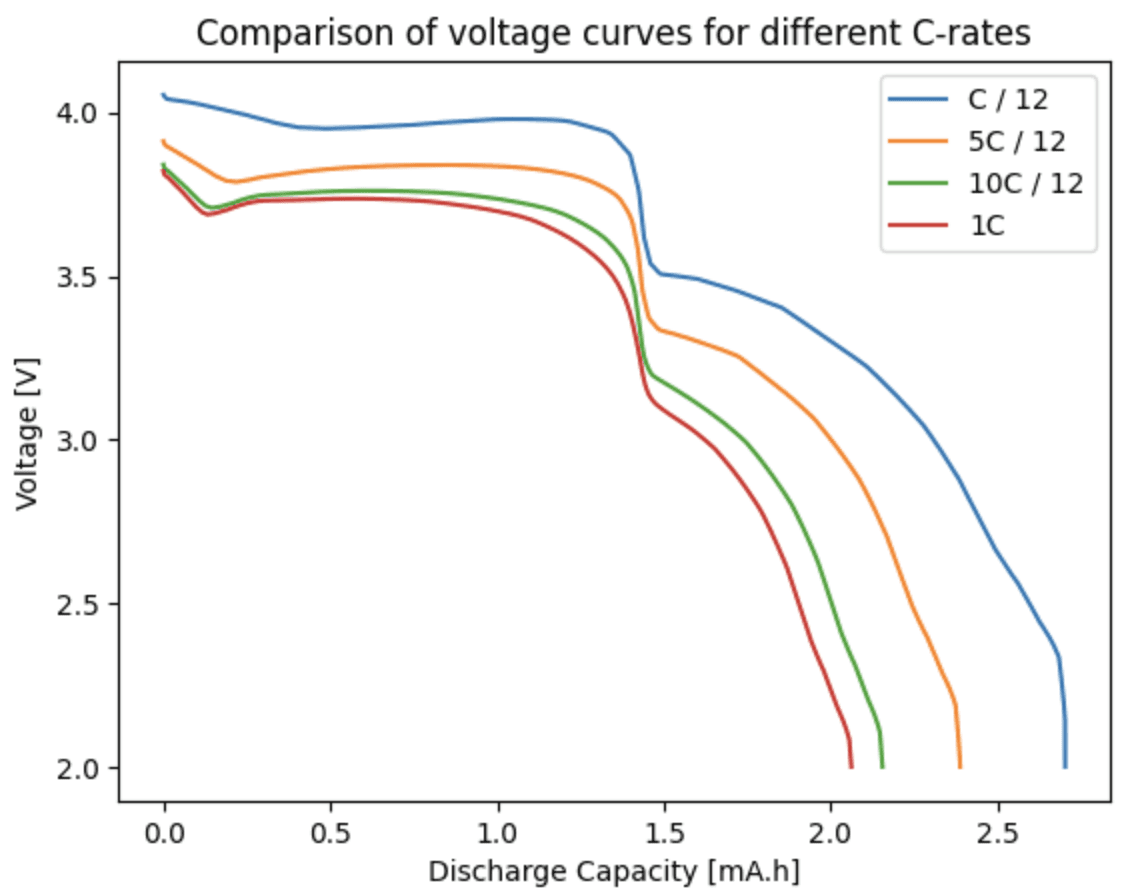
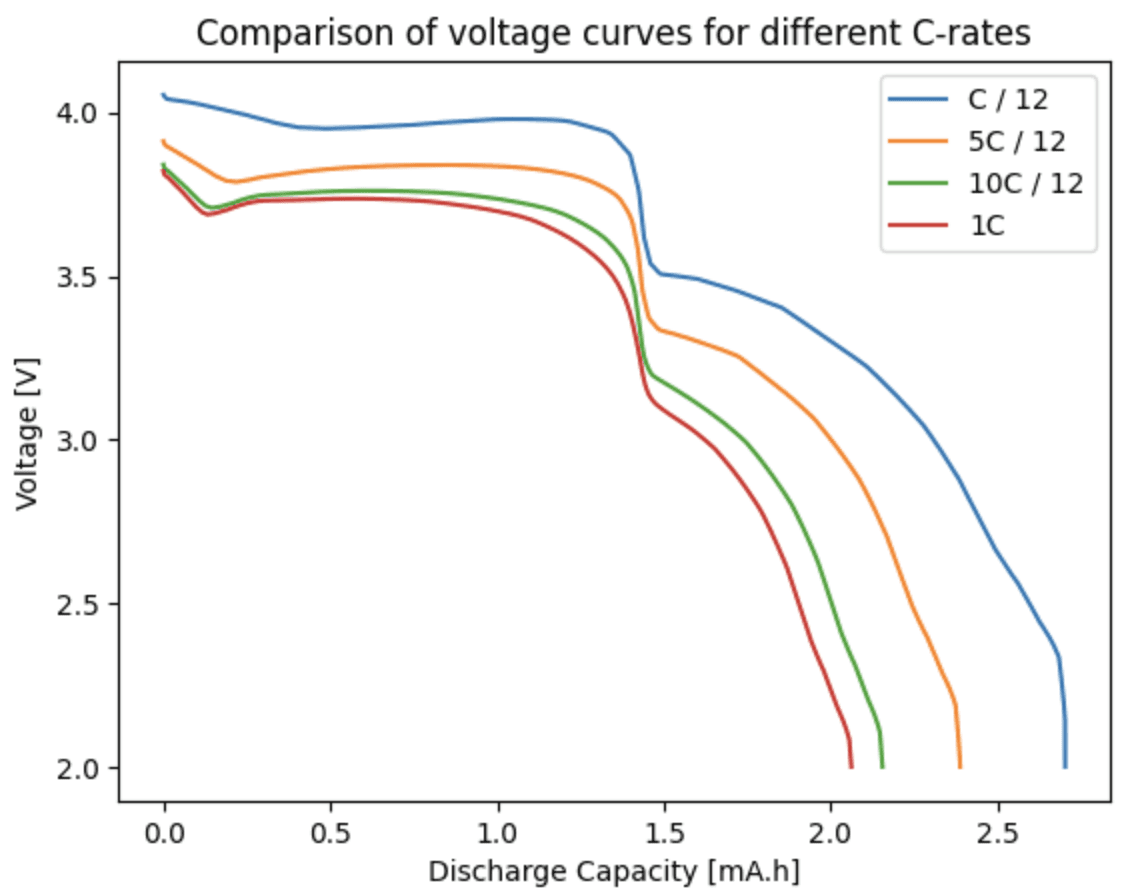
Sodium Ion Battery Model now available in PyBaMM!
This blog post explores the history of SIBs, the intricacies of the new PyBaMM model, and the exciting possibilities it unlocks for the future of energy storage.
Nov 12, 2024
Run your first virtual battery test today
Simulate, iterate, and validate your cell configurations with no lab time required.
Ionworks Technologies Inc. All rights reserved.
Run your first virtual battery test today
Simulate, iterate, and validate your cell configurations with no lab time required.
Run your first virtual battery test today
Simulate, iterate, and validate your cell configurations with no lab time required.
Ionworks Technologies Inc. All rights reserved.
Run your first virtual battery test today
Simulate, iterate, and validate your cell configurations with no lab time required.
Ionworks Technologies Inc. All rights reserved.